Revolutionizing Electric Vehicles: Next-Gen Battery Tech
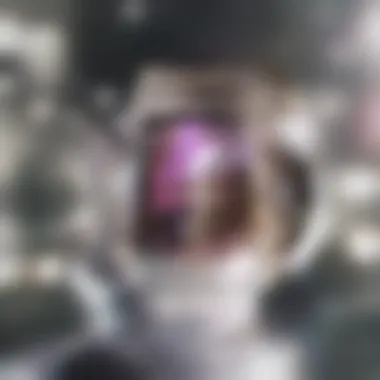
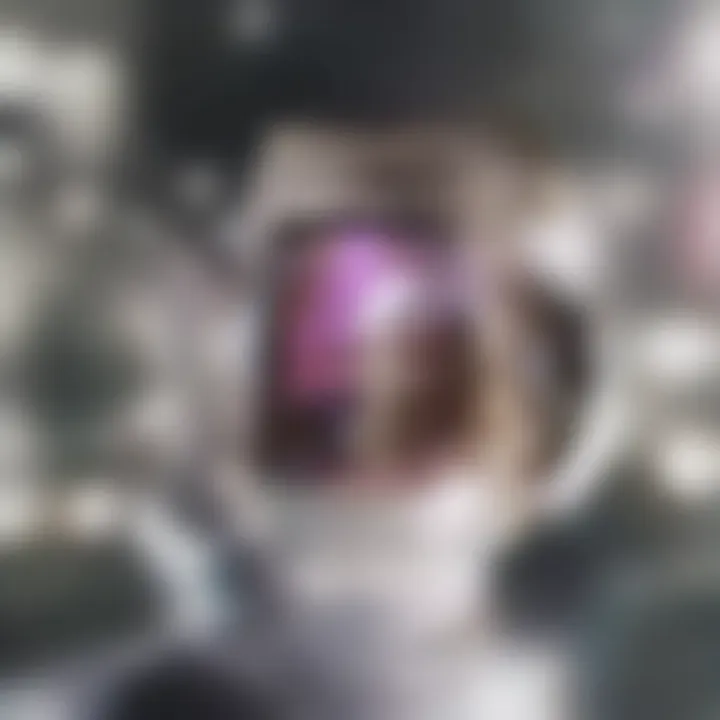
Intro
The urgency for innovative battery technologies in electric vehicles (EVs) cannot be overstated. As industries and consumers alike emphasize the need for more sustainable and longer-lasting energy solutions, research and development in battery technology are gaining momentum. This demand drives innovation and creates an opportunity for transformative changes to occur in the EV sector.
Various emerging battery technologies are underway, prominently including solid-state batteries and lithium-sulfur batteries. Each of these technologies presents unique advantages and challenges. Stakeholders from various industries are investing heavily to understand and harness these new advancements effectively. This article will explore the key components of this evolving landscape, looking closely at the significant changes that might influence future EV markets.
Summary of Objectives
The primary objective of this article is to investigate new battery technologies that could substantially improve electric vehicle performance. By scrutinizing advancements like solid-state batteries, we aim to clarify how these innovations can reshape the current automotive landscape.
Importance of the Research
Sustainability and efficiency are vital in todayβs world, particularly regarding energy use. Consequently, understanding new battery technologies is not only relevant but essential. It allows researchers, policymakers, and industry leaders to adapt to emerging trends that can affect energy consumption and environmental impacts. Insights from this research can guide strategic decisions in technology development and regulatory measures.
Results and Discussion
Presentation of Findings
In our examination of the battery technology advancements, notable findings include:
- Solid-state batteries promise greater energy density and safety compared to traditional lithium-ion systems.
- Lithium-sulfur technology offers potential cost advantages and lower environmental impact due to the abundance of sulfur.
- Key players in this space include auto manufacturers and technology companies highly invested in R&D.
These findings indicate a clear trend toward more efficient, safer, and environmentally friendly battery technologies.
Implications of Results
The implications of these results are profound. As battery technology evolves, we expect significant shifts, including:
- Improved performance metrics for EVs, leading to increased consumer adoption.
- Enhanced safety standards, potentially reducing the rate of battery-related incidents.
- Environmental benefits from using less harmful materials and effective recycling processes.
"The transition to advanced battery technologies represents not only a technological leap but also a commitment to sustainable practices in the automotive industry."
The exploration of these new technologies will enhance knowledge and contribute to better policy-making and investment strategies moving forward.
Preface to Electric Vehicle Battery Technology
The evolution of electric vehicle battery technology is critical in shaping the future of sustainable transportation. As the demand for electric vehicles grows, understanding how these power systems function becomes essential. Battery technology not only defines the driving range and charging speed of electric vehicles but also influences safety and environmental impacts. Therefore, an in-depth exploration of battery systems is not just beneficial; it is necessary to grasp the challenges and developments ahead.
Overview of Current Battery Technologies
Currently, lithium-ion batteries dominate the electric vehicle market. Their efficiency and performance have led to widespread implementation. However, new technologies are on the horizon. These include solid-state batteries, lithium-sulfur batteries, and graphene-based batteries. Each carries its own advantages and drawbacks.
- Lithium-Ion Batteries: Known for their energy density and longevity.
- Solid-State Batteries: Offer higher safety and energy density potential.
- Lithium-Sulfur Batteries: Potential for higher capacity at a lower cost.
The key to advancing EV technology lies in both improving these existing systems and exploring novel alternatives.
Importance of Advancements in Battery Technology
Advancing battery technology is of utmost importance for the electric vehicle sector. New developments aim to enhance several aspects:
- Energy Density: Increasing the amount of energy stored in a given space improves vehicle range.
- Charging Times: Quicker charging capabilities enhance user convenience and accessibility.
- Safety: With incidents of battery fires becoming a concern, innovations in safety features are paramount.
- Sustainability: Environmentally friendly materials and processes can reduce the overall ecological footprint of battery production and disposal.
Experts argue that these advancements will not only boost electric vehicle adoption but also align with global sustainability goals.
"Innovations in battery technology are pivotal for overcoming the barriers to widespread electric vehicle use."
As the industry progresses, it becomes clear that the future of transportation will heavily rely on ongoing research and improvements in battery technology. Understanding these nuances is vital for anyone involved in the field.
Solid-State Batteries
Solid-state batteries represent a key advancement in battery technology, particularly for electric vehicles. These batteries, which utilize solid electrolytes instead of the liquid or gel electrolytes found in traditional lithium-ion batteries, promise to significantly enhance performance, safety, and longevity. Understanding solid-state batteries is essential, as they could ultimately lead to safer, more efficient, and longer-lasting electric vehicles, addressing many of the current limitations faced by the automotive industry.
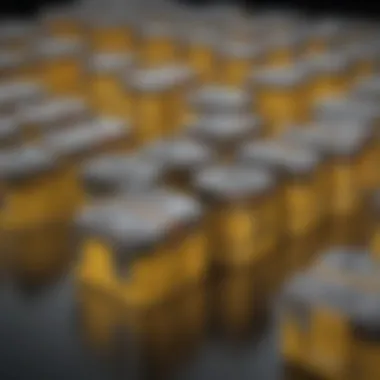
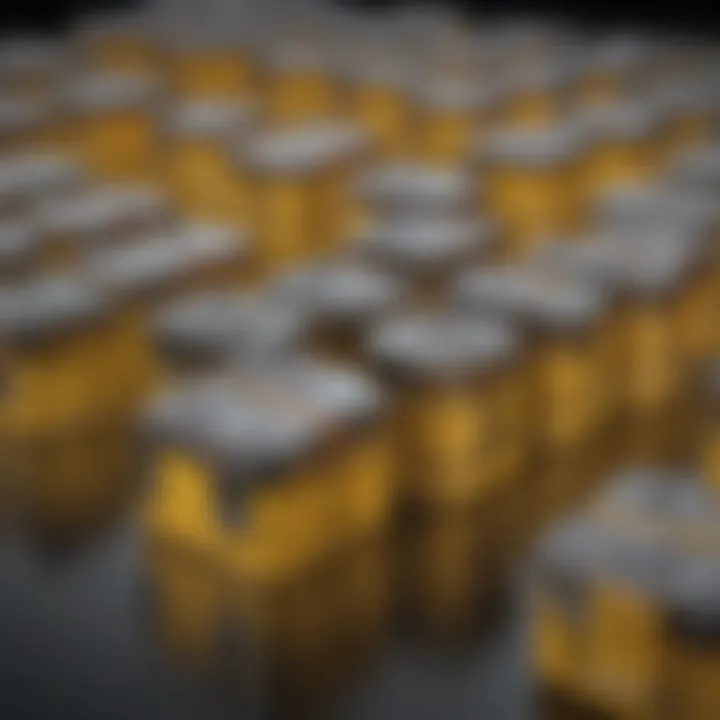
Technical Overview
A solid-state battery consists mostly of a solid electrolyte, which facilitates ion movement between the battery's cathode and anode. Unlike conventional batteries, the solid electrolyte does not leak and is less prone to thermal runaway. The common materials used for solid electrolytes include ceramics and certain polymers, each offering distinct advantages. The design promotes a higher energy density, enabling electric vehicles to travel further on a single charge. Energy density is vital for improving the overall efficiency of EVs, making solid-state technology an attractive option.
Advantages Over Lithium-Ion Batteries
Solid-state batteries come with several notable advantages compared to traditional lithium-ion batteries:
- Greater Energy Density: Higher capacity allows for increased driving range.
- Enhanced Safety: The likelihood of leakage and fires is reduced, minimizing hazards associated with battery malfunctions.
- Longer Lifespan: Solid-state batteries show potential for a longer cycle life, which means they would not need to be replaced as frequently.
- Wide Operating Temperature Range: These batteries can function in extreme temperatures better than their counterparts.
These benefits position solid-state batteries as a possible solution to some of the ongoing challenges in electric vehicle performance and safety.
Challenges in Commercialization
Despite their advantages, the path to commercialization of solid-state batteries is fraught with challenges:
- Manufacturing Complexity: Producing solid-state batteries at scale involves complex processes, which can drive up costs significantly.
- Material Limitations: Finding suitable materials that are both conductive and stable has proven to be difficult, affecting development timelines.
- Integration Issues: Integrating solid-state technology into existing vehicle architectures poses additional engineering challenges.
As manufacturers work to overcome these obstacles, the timeline for widespread adoption remains uncertain. However, ongoing research is actively addressing these issues, with the potential for breakthroughs that could expedite the introduction of solid-state batteries to the market.
Lithium-Sulfur Batteries
Lithium-sulfur batteries are becoming increasingly significant in the landscape of electric vehicle technology. They offer promising alternatives to conventional lithium-ion batteries, primarily due to their high energy density, which has the potential to enhance the range of electric vehicles. Understanding the specific elements that constitute lithium-sulfur batteries is crucial for comprehending their benefits and the associated challenges that come with their adoption.
Chemical Composition and Functionality
Lithium-sulfur batteries operate on a fundamentally different chemistry compared to traditional lithium-ion batteries. They consist of a lithium anode and a sulfur-based cathode. The sulfur has a high theoretical capacity, which allows these batteries to store more energy than lithium-ion types. When the battery discharges, lithium ions move from the anode to the cathode, undergoing conversion through a series of lithium polysulfide intermediates. This process significantly affects their efficiency and overall performance.
However, the increasing solubility of polysulfides in lithium-sulfur batteries is a notable issue. It can lead to what is termed "shuttle effect," which reduces the battery's overall life and efficiency. Therefore, ongoing research in the field aims at improving the containment of these polysulfides to enhance battery longevity and stability.
Potential Benefits for Electric Vehicles
The advantages of lithium-sulfur batteries over conventional technologies are multifaceted. Key benefits include:
- Higher Energy Density: Lithium-sulfur batteries can theoretically achieve up to five times the energy density of lithium-ion batteries, potentially leading to a substantial increase in driving range for electric vehicles.
- Lightweight: Sulfur is abundant and inexpensive compared to transition metal oxides used in lithium-ion batteries, which makes lithium-sulfur batteries cost-effective and potentially lighter, aiding in vehicle efficiency.
- Environmentally Friendly Materials: The environmental impact is of growing concern in battery production. Sulfur is non-toxic and abundant, which aligns with wider sustainability goals within the industry.
These benefits position lithium-sulfur chemistry as a serious contender in the future of electric vehicle batteries, especially in applications where weight and energy density are critical.
Research and Development Efforts
Current research into lithium-sulfur batteries is focused on addressing their inherent limitations and optimizing their performance for commercial use. Key areas of investigation include:
- Polysulfide Management: Scientists are exploring various strategies, including using modified cathodes and additives to mitigate polysulfide dissolution.
- Electrode Design: Innovation in electrode structures is crucial. For example, incorporating conductive materials into sulfur electrodes can improve conductivity and enhance overall efficiency.
- Lifecycle Improvements: Studies focus on understanding the cycling stability and longevity of lithium-sulfur batteries, with the goal of achieving a lifespan comparable to that of conventional batteries.
Overall, the ongoing advances in research are promising and aim at unlocking the full potential of lithium-sulfur batteries. This technology may play a significant role in the future of energy storage for electric vehicles, but it requires careful navigation through its current challenges to reach commercial viability.
"The transition to lithium-sulfur battery technology could significantly influence the dynamics of the electric vehicle market, reshaping how we think about vehicle range and efficiency."
By investing in research and fostering innovative developments, the path toward integrating lithium-sulfur batteries into the mainstream electric vehicle framework appears increasingly attainable.
Graphene-Based Batteries
Graphene-based batteries are at the forefront of emerging energy storage technologies, offering unique advantages that could redefine electric vehicle (EV) performance. This section will outline the properties of graphene, its specific applications in electric vehicles, and discuss the current limitations contrasted with future prospects.
Properties and Benefits of Graphene
Graphene, a single layer of carbon atoms arranged in a hexagonal lattice, exhibits remarkable properties. It is known for its excellent electrical conductivity, mechanical strength, and thermal properties. Some of the key benefits of graphene-based batteries include:
- High Conductivity: Graphene facilitates rapid electron movement, which can significantly improve battery charging and discharging rates.
- Lightweight: The structure of graphene is incredibly light, which can reduce the overall weight of the battery, enhancing vehicle efficiency.
- Durability: Graphene's strength contributes to longer battery lifespans and higher cycle stability, making it less prone to degradation.
- Environmental Impact: As a more abundant material than the metals used in traditional batteries, graphene supports sustainable practices in battery production.
These properties make graphene an appealing alternative to conventional lithium-ion batteries, offering the potential for faster charging times and longer ranges for electric vehicles.
Applications in Electric Vehicles
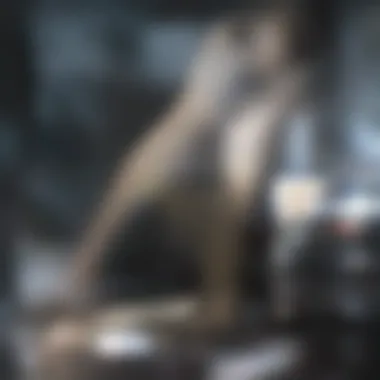
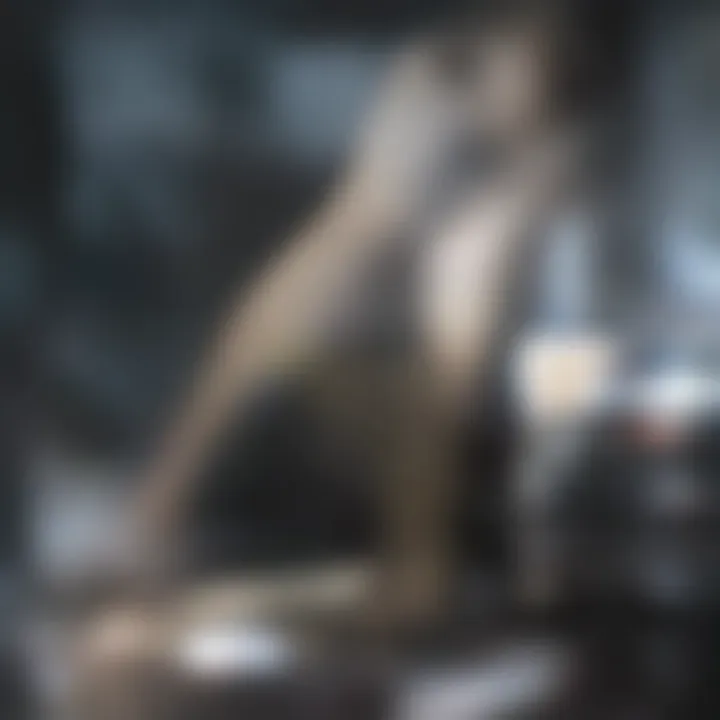
Graphene can be incorporated into battery technology in various ways. Here are some notable applications:
- Graphene-Oxide Batteries: These batteries utilize graphene oxide as a component, enhancing charge storage capacity while maintaining stability. This can lead to improved energy density compared to organic lithium batteries.
- Supercapacitors: Graphene supercapacitors offer a hybrid storage solution that combines high power density with some energy storage capabilities. They enable fast bursts of energy, such as during acceleration.
- Hybrid Designs: Some research is exploring hybrid batteries that blend graphene with established systems to capitalize on the strengths of both materials.
By leveraging grapheneβs unique characteristics, manufacturers can create batteries that not only charge faster but also last longer and perform better under diverse conditions.
Current Limitations and Future Prospects
Despite the capabilities of graphene-based batteries, several challenges remain before widespread adoption can occur. Limitations include:
- Manufacturing Costs: The production of high-quality graphene remains expensive. This issue hinders its commercial viability for mass-market applications.
- Scalability: While lab-scale successes exist, scaling the production of graphene for commercial batteries poses significant technical challenges.
- Integration: Integrating graphene into existing battery systems requires further research to fully understand compatibility and performance dynamics.
Nevertheless, the future prospects for graphene in battery technology appear promising. Ongoing research projects aim to develop cost-effective manufacturing processes, while advancements in materials science may pave the way for more efficient use of graphene in batteries.
In summary, as the pursuit of more efficient and sustainable battery technologies continues, graphene-based systems may play a major role in the next generation of electric vehicle batteries.
Battery Management Systems
Battery Management Systems (BMS) play a vital role in the efficiency and longevity of electric vehicle (EV) batteries. Thses systems are central to ensuring the optimal performance of battery packs. By monitoring health, controlling charging and discharging, and providing safety measures, BMS significantly contribute to enhancing the overall user experience of EVs. This section delves into the specific elements that define the function and significance of BMS in the realm of electric vehicle battery technology.
Role in Electric Vehicles
The primary function of a Battery Management System is to act as the brain of the battery pack. It collects critical data from individual cells within the battery, helping to ensure that each cell operates within its safe limits. An important aspect of this include:
- Monitoring Voltage and Temperature: BMS constantly oversees the voltage levels of each cell. This is essential since overcharging can lead to battery failure. It also monitors the temperature, as extreme heat can degrade battery performance.
- Balancing Cell Charges: It ensures that all cells within a battery pack charge and discharge uniformly. Uneven charge levels can lead to reduced battery life and efficiency.
- Safety Features: BMS incorporates safety protocols to instantly disconnect the battery if it detects potential issues, like short circuits or extreme temperatures. This is crucial for enhancing user safety.
The efficiency of an EV battery hinges on effective management systems. Advanced BMS can directly influence how long an EV can run between charges, making this a critical topic for both manufacturers and consumers.
Integration with New Battery Technologies
As new battery technologies emerge, the adaptability of BMS becomes increasingly important. For instance, with the advent of solid-state and lithium-sulfur batteries, BMS must evolve to address the unique characteristics of these technologies. Some key considerations include:
- Compatibility with Advanced Chemistry: The BMS needs to adjust to the different voltage and capacity profiles of new battery types. This increases the complexity and requires sophisticated algorithms.
- Scalability: With large battery packs, especially in electric buses or trucks, BMS must manage a greater number of cells. Scaling BMS appropriately is crucial to maintain safety and performance.
- Data Analytics: The rise of smart grid technology allows BMS to utilize data analytics for predictive maintenance. This can enhance battery lifespan and performance metrics, ultimately benefiting the end user.
"The integration of Battery Management Systems with advanced battery technologies plays a pivotal role in establishing the reliability and efficiency that is needed in electric vehicles today."
As the EV industry continues to innovate, the role of BMS will remain central to maximizing the potential of new battery technologies. By ensuring safe operation and long-term performance, efficient BMS will support the transition to a sustainable transportation future.
The Role of Recycling in Battery Sustainability
The recycling of batteries plays a crucial role in enhancing the sustainability of electric vehicle technologies. As the demand for electric vehicles continues to grow, so does the need for efficient waste management practices regarding batteries. These components contain various materials that can be hazardous if improperly disposed of, such as lithium, cobalt, and nickel. Recycling helps to mitigate environmental impact and ensures that valuable materials can be recovered and reused. This is increasingly important as global initiatives push towards eco-friendly solutions in transportation.
Efficient recycling processes not only reduce the amount of waste directed to landfills but also contribute significantly to conserving resources. By reclaiming metals and chemicals from spent batteries, manufacturers can minimize dependence on mining, which is often environmentally damaging. Moreover, effective recycling lowers production costs for new batteries, presenting economic benefits alongside environmental gains.
Current Recycling Methods
The current battery recycling methods can be categorized primarily into pyrometallurgical and hydrometallurgical processes.
- Pyrometallurgical recycling involves high-temperature processing to extract metals from batteries. This method is efficient, but it requires significant energy input, resulting in a higher carbon footprint.
- Hydrometallurgical recycling uses aqueous solutions to leach metals from batteries. This method is generally more environmentally friendly, but it may not recover all types of materials effectively.
Additionally, several companies are developing innovative techniques, such as direct recycling. This involves reusing electrodes from used batteries without needing complete dismantling, preserving the cleanliness and integrity of materials. Companies like Li-Cycle and American Battery Technology Company are pioneering these newer methods to enhance sustainability practices in the sector.
Future Innovations in Recycling Technology
Looking ahead, the battery recycling sector is on the brink of several significant innovations that could reshape the landscape. One key area of development is the advancement of closed-loop systems. This approach aims to create a cycle where battery materials are continuously reclaimed and reintegrated into the production cycle, directly reducing the need for newly mined resources.
Another potential innovation includes improvements in sorting technology that enhance the efficiency at which recycled materials are processed. Automated methods and machine learning could streamline the identification and sorting of battery components, maximizing material recovery rates.
Furthermore, as the technologies for electric vehicles evolve, recycling processes will likely adapt to accommodate new materials and battery chemistries. Innovations in biotechnology, for instance, might enable the use of biological processes for metal recovery, offering a more sustainable alternative to traditional methods.
In summary, the recycling of batteries is pivotal for sustaining electric vehicle technologies. As we progress, ongoing research and innovation will play essential roles in enhancing efficiency while reducing environmental footprint. Emphasizing recycling in battery development aligns closely with the broader goal of creating a more sustainable future, ensuring that the electric vehicle market can meet growing demands responsibly.
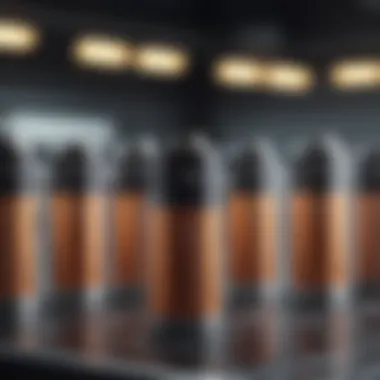
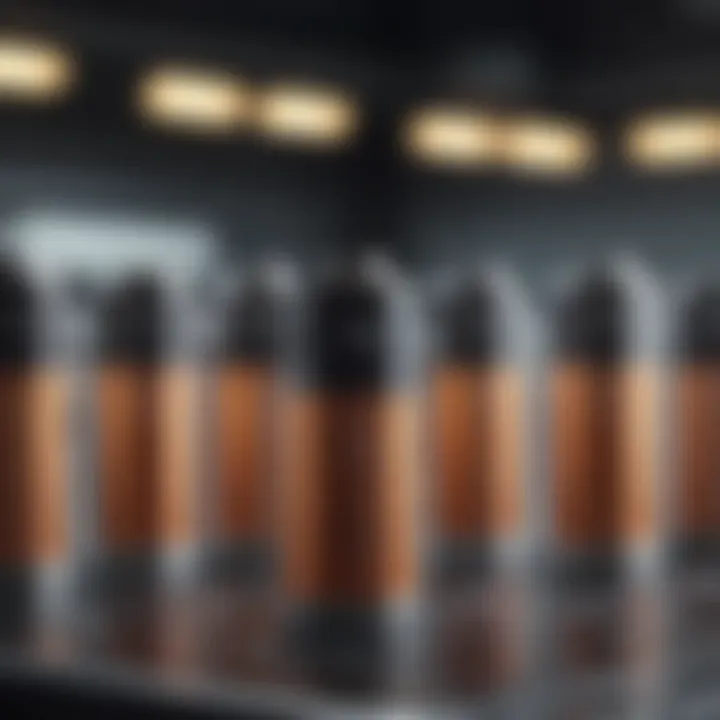
"Effective battery recycling is not just a necessity; it's an integral part of the sustainability cycle in electric vehicle technology."
Policy and Regulatory Considerations
The landscape of electric vehicle (EV) battery technology is significantly influenced by policy and regulatory factors. Individuals and companies investing in new battery technologies need to navigate a complex web of laws and guidelines that can either facilitate growth or stifle innovation. Effective policy frameworks can lead to rapid advancements, while stringent regulations can hinder access to necessary resources.
Incorporating well-designed policies that support sustainable practices can benefit both the environment and the economy. Governments can stimulate research and development through grants and tax incentives, which are crucial for organizations pushing the boundaries of battery technology. Such incentives not only promote progress but also help mitigate the risks associated with high-metal dependency and environmental impacts.
Additionally, regulatory considerations ensure that new technologies meet safety standards and environmental requirements. This includes the assessment of materials used in batteries and their end-of-life disposability. Policies can guide the responsible sourcing of materials, reducing harm to ecosystems and promoting recycling initiatives.
"Robust regulatory frameworks are essential in promoting innovation while ensuring safety and sustainability in the growing EV industry."
Government Incentives for New Technologies
Government incentives play a vital role in the evolution of battery technologies for electric vehicles. These incentives can include tax breaks, subsidies, grants, and favorable loan arrangements aimed at encouraging research and market growth. Companies developing solid-state batteries or lithium-sulfur technology, for instance, can benefit from financial support that reduces their operational risks and promotes further innovation.
Some important factors related to government incentives include:
- Tax Credits: Offering tax credits for R&D in battery technology encourages companies to invest more in innovative processes.
- Subsidies for Production: Financial assistance can lower the cost of manufacturing advanced battery types, making electric vehicles more affordable to consumers.
- Investment in Infrastructure: Government funding for charging stations and battery recycling facilities can further enhance the growth of electric vehicles.
The implications of such incentives can be profound, leading to higher adoption rates of electric vehicles and encouraging sustainable habits among consumers.
Global Regulations Impacting Battery Development
Global regulations have a significant impact on the direction of EV battery technology. Various international agreements and national regulations set standards for safety, efficiency, and environmental protection. As electric vehicles become more prevalent, regulatory bodies are focusing on ensuring that battery technologies do not compromise human health or the environment.
Some key points about global regulations include:
- Safety Standards: Organizations such as the International Electrotechnical Commission (IEC) set safety standards that all battery technologies must meet to protect users and manufacturers alike.
- Environmental Regulations: These regulations emphasize the importance of sustainable practices in the extraction of materials and the disposal of batteries. Compliance with these regulations is crucial for companies aiming to market their technologies globally.
- Trade Policies: Tariffs and trade agreements can influence the supply chain of battery materials. Favorable trade policies can reduce costs and improve access to critical resources needed for battery production.
Staying aware of these regulatory frameworks will help developers align their innovations with international standards, ultimately paving the way for safer, more efficient battery technologies.
Culmination and Future Outlook
The advancements in battery technology are critical for the success and sustainability of electric vehicles. As the demand for clean transportation increases, it is essential that we focus on creating batteries that not only enhance vehicle performance but also reduce environmental impact. This section synthesizes key insights from the article and provides a forward-looking view on how battery technology will evolve in the coming years.
Overview of Industry Trends
The electric vehicle industry is experiencing significant growth driven by several trends. First, the shift toward sustainable solutions is accelerating. Innovators are not only focused on improving capacity and efficiency, but also on ensuring that materials used in batteries are more sustainable.
Second, collaboration across sectors has become a hallmark of this evolution. Companies are partnering with researchers and academic institutions to share findings and accelerate developments.
- Increased investment in research and development is crucial. Firms like Tesla and Toyota are redefining their approaches, exploring solid-state batteries and lithium-sulfur chemistry.
- Market expansion is another factor. More automakers are entering the electric vehicle market, increasing competition and driving innovation.
- Consumer awareness of battery performance and environmental impact is growing, pressuring manufacturers to adopt greener practices.
Anticipated Developments in Battery Technology
Looking ahead, several promising developments are on the horizon. One area is the advancement of solid-state batteries, which are praised for their potential in offering higher energy densities and improved safety features compared to traditional lithium-ion batteries.
Another area with promise is lithium-sulfur technology. It is expected to enhance range and reduce costs. Research continues to eliminate key barriers, such as cycle life.
Additional anticipated developments include:
- Enhanced battery management systems that leverage artificial intelligence for better performance and longevity.
- Recycling technologies that recover valuable materials from old batteries, promoting sustainability.
- Solid-state designs becoming commercially viable, impacting mass production and pricing in the market.
The future of battery technology is not just about better batteries but also about integrating these advancements into a holistic and sustainable transport ecosystem.
As these innovations unfold, the intersection of battery technology with renewable energy sources will further transform the electric vehicle landscape, making it more integrated and environmentally friendly. This evolution is not only crucial for the automotive sector but is also pivotal in fostering a greener future.
Citations and Academic References
To offer readers a robust understanding of battery technology, this section lists out key academic references and citations used throughout the article. Engaging with these sources can deepen oneβs grasp on the subject matter. Such references might include academic journals on energy storage, publications discussing solid-state batteries, lithium-sulfur advancements, and broader analyses on the sustainability of battery technologies.
Additionally, keeping abreast of ongoing studies and innovations is crucial. Many significant advancements are documented in journals like Journal of Power Sources or Energy Storage Materials. Being thoroughly informed about these documents will serve the reader well.
An ordered list of suggested references might look like this:
- Journal of Power Sources
- Energy Storage Materials
- Reports by battery manufacturers like CATL and LG Chem.
- White papers on solid-state battery technology from research institutions.
- Articles from industry analysis platforms analyzing future trends in battery technology.
By evaluating these references, readers can better appreciate the context and the evolution of battery technologies in electric vehicles.