Exploring the Science of Thin Film Polarizers
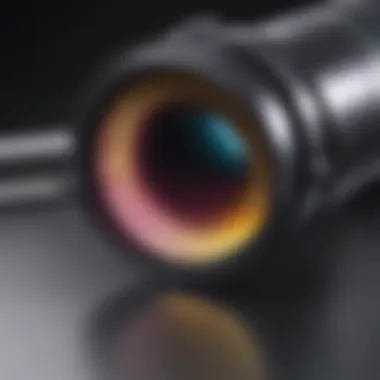
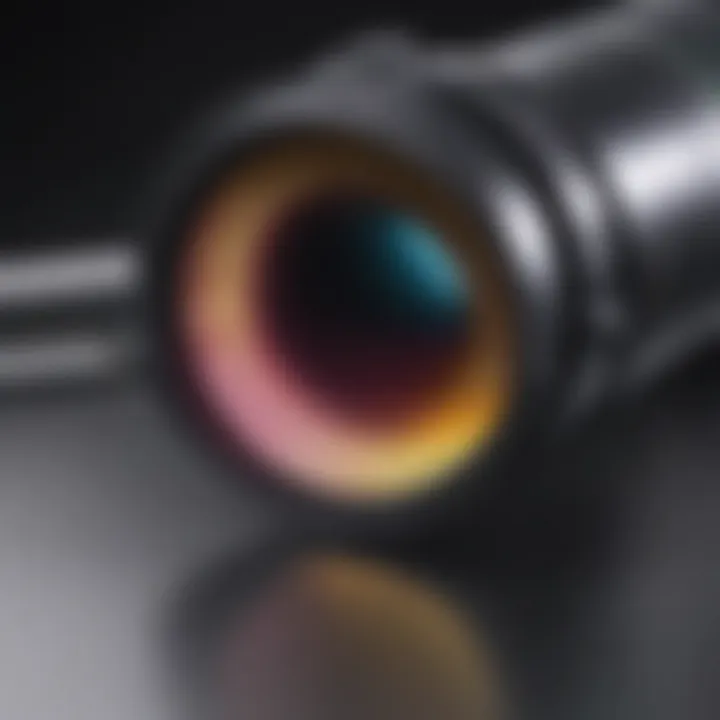
Summary of Objectives
This article provides a thorough examination of thin film polarizers, elucidating their essential principles, design strategies, and applications in numerous fields. Emphasis will be placed on the underlying physics, fabrication methods, and the broad spectrum of uses in optical technologies. Our goal is to create a resource that enlightens researchers, educators, and professionals regarding the importance of thin film polarizers and the evolution in this realm.
Importance of the Research
Thin film polarizers play a critical role in the field of optics. Given their applications in telecommunications, imaging systems, and display technologies, understanding these components can lead to pivotal advancements. With continuous innovations, the study of thin film polarizers becomes increasingly relevant, providing a foundation for future optical developments.
Intro
Thin film polarizers are integral components in modern optical systems, offering a means to manipulate light in specific ways. These devices utilize the principles of interference and selective absorption to achieve polarization, which is crucial in various applications. Researchers and professionals alike recognize the importance of these polarizers in enhancing the performance of optical devices, such as cameras and projectors.
The journey into the realm of thin film polarizers begins with a look at their basic workings. By examining how these films are constructed, we gain insights into their operational effectiveness. Next, we analyze the various material compositions used in their manufacture. This article aims to reveal not only the technicalities of thin film polarizers but also their applications and relevance within todayβs technological landscape.
Results and Discussion
Presentation of Findings
The findings discussed in this article showcase how thin film polarizers stand out in diverse applications. For instance, high-performance polarizers are crucial in liquid crystal displays where image clarity is paramount. Recent developments reveal advancements in thin film technology, particularly concerning their transmission efficiency and durability.
Thin film polarizers have evolved significantly, gaining importance in emerging fields such as augmented and virtual reality.
This growth is evident in industries ranging from telecommunications to medical imaging, where precise light control greatly enhances functionality.
Implications of Results
The implications of this research are multi-faceted. The advancements in thin film polarizer technology could enhance the efficiency of optical systems, leading to greater performance in various devices. As industries adopt these innovations, we may see fundamental changes in how optical technologies are implemented and accessed.
By unpacking the complexities of thin film polarizers, we prepare the ground for future innovations while inspiring further research in optical sciences.
Understanding these components not only aids in the current technological context but also shapes future applications and designs.
Preface to Thin Film Polarizers
Thin film polarizers play a crucial role in the field of optics, greatly enhancing the functionality of various optical systems. These components selectively filter light, allowing only waves vibrating in a specific direction to pass through. This property is essential in applications ranging from photography to telecommunications. The intricate nature of light polarization makes thin film polarizers not just a simple tool, but a sophisticated solution to numerous optical challenges.
Understanding thin film polarizers involves exploring their definition, basic principles, and historical context. This foundational knowledge sets the stage for delving into more complex topics related to their design and applications.
Definition and Basic Principles
Thin film polarizers are optical devices constructed from multiple layers of dielectric materials. These layers are often applied to a substrate through advanced manufacturing techniques, tailoring the film's thickness to specific wavelengths of light. The essential working principle behind these polarizers lies in their ability to exploit the phenomena of interference and birefringence.
The interference effect occurs when light waves reflecting off the different layers of the film interact, leading to constructive and destructive interference. This interaction enhances or diminishes light intensity depending on its polarization state. Furthermore, birefringence is a property of certain materials that causes them to refract light differently based on polarization direction. This dual mechanism enables thin film polarizers to efficiently separate polarized light from unpolarized light.
Historical Development
The evolution of thin film polarizers is deeply rooted in advancements in optical technology. The groundwork for modern thin film polarizers began in the 19th century, with early studies on light polarization by scientists such as Γtienne-Louis Malus and Augustin-Jean Fresnel. Their work laid the foundation for understanding how light waves interact with materials.
In the mid-20th century, technological progress led to the emergence of thin film deposition techniques, significantly enhancing the production of polarizers. Physical Vapor Deposition (PVD) and Chemical Vapor Deposition (CVD) became standard methods, allowing for precise control over film thickness and quality.
Today, research continues to push the boundaries of thin film polarizer technology. Innovations in nano-structured materials and advanced manufacturing techniques are enabling the development of more efficient and versatile polarizers, crucial for modern applications in imaging, display technologies, and communication systems.
Optical Principles Behind Polarization
Understanding the optical principles behind polarization is fundamental to grasping how thin film polarizers function. Polarization refers to the orientation of light waves in relation to their direction of travel. In various scientific and engineering applications, it becomes essential to control light characteristics. Polarizers selectively transmit specific orientations of light waves while blocking others, making them crucial in optical devices. These principles form the foundation upon which the technology surrounding thin film polarizers is built.
Understanding Light Polarization
Light commonly interacts with materials that can modify its characteristics. The wave nature of light leads to different polarization states. A fundamental aspect is that natural light contains waves vibrating in multiple directions. When light hits a surface or passes through a polarizing filter, its vibrational direction can change. This can lead to either a change in intensity or a complete blockage of certain light waves. Knowing how this works helps in designing devices that efficiently utilize polarized light.
Types of Polarization
There are various types of polarization, each with unique characteristics. This distinction allows tailored applications in different fields.
Linear Polarization
Linear polarization is when light waves vibrate in a singular direction. It is commonly used in many optical applications due to its simplicity and effectiveness. The key characteristic of linear polarization is its ability to efficiently reduce glare, which can be beneficial in photography and visual displays. Its unique feature is that it can be achieved through a variety of methods such as reflection or absorption. However, the main disadvantage is that it typically only blocks light in the perpendicular orientation, making it less adaptable in situations requiring multi-directional polarization.
Circular Polarization
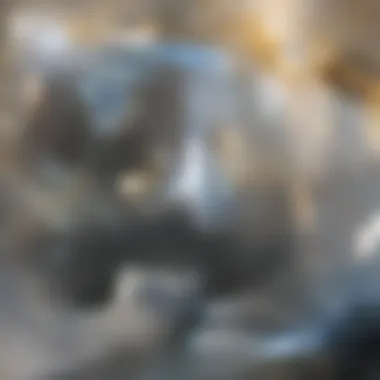
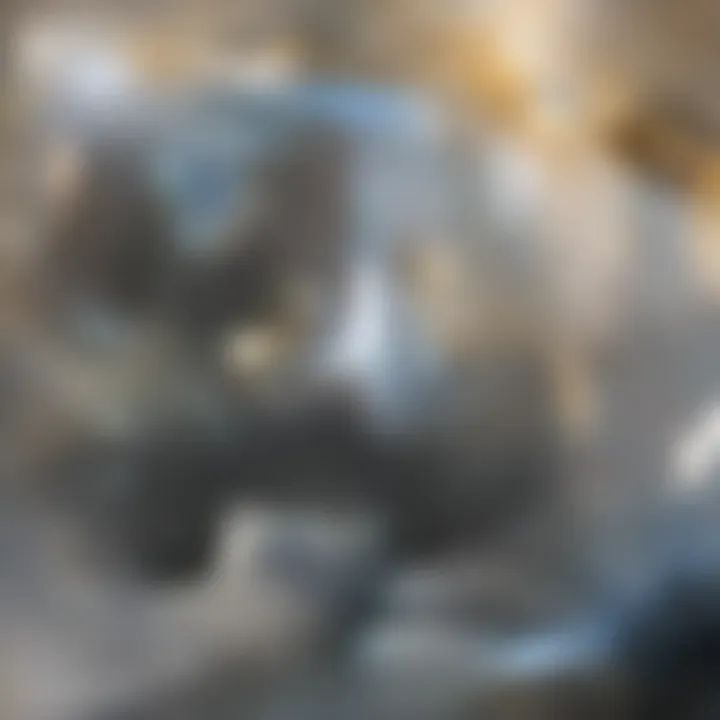
Circular polarization involves light waves rotating as they propagate. This polarization type can be advantageous in 3D imaging and optical communication systems. The key characteristic is the ability to maintain intensity regardless of the angle of observation. This makes circular polarizers a popular choice for screens and cameras. However, one significant disadvantage is that they generally require more complex optical setups, which might raise manufacturing costs.
Elliptical Polarization
Elliptical polarization is a generalized case of circular polarization. Here, the electric field of light traces an ellipse, presenting a combination of linear and circular characteristics. Its importance lies in its flexibility; it can represent both linear and circular polarizations. A significant characteristic of elliptical polarization is that it can be tuned to specific applications. However, it might complicate the design of polarizing filters, especially when manufacturing processes are considered.
"Understanding the different types of polarization is essential for designing effective optical devices. Each type presents unique advantages and constraints."
By acknowledging these types of polarization, one can better comprehend the applications and limitations of thin film polarizers in various optical technologies.
Design Architecture of Thin Film Polarizers
The design architecture of thin film polarizers is crucial for their performance and application. Understanding this architecture allows researchers and professionals to optimize polarizer efficiency and functionality. The arrangement of layers and the choice of materials directly influence the optical properties of these devices. Ultimately, effective design can enhance the usability in various fields, such as telecommunications and imaging systems.
Layer Structure and Materials Used
Dielectric Materials
Dielectric materials form the primary layers in thin film polarizers. They are non-conductive, which allows them to support electric fields without conducting electricity. A key characteristic of dielectric materials is their high refractive index, enabling significant control over light behavior. They are highly beneficial in designing polarizers as they can be engineered to achieve desired polarization effects.
One unique feature of dielectric materials is their ability to minimize optical losses while maintaining durability and stability. Their resistance to environmental factors enhances the longevity of polarizers, which is an important advantage in practical applications. However, the production process can be sensitive, and quality control is essential to achieve optimal performance.
Metallic Coatings
Metallic coatings play a significant role in enhancing the reflective properties of thin film polarizers. Typically used as the top or bottom layers of polarizer architecture, metallic coatings are conductive films that facilitate the selective reflection of specific light wavelengths. A key characteristic is their ability to provide high reflectivity for certain polarization directions, which is essential in optical applications.
Metallic coatings may offer improved thickness control, which allows for precise adjustments tailored to the specific requirements of a device. However, one disadvantage is their susceptibility to oxidation, which can degrade performance over time. Managers in optical engineering must consider this when choosing materials and techniques for manufacturing.
Anti-Reflection Coatings
Anti-reflection coatings are essential in minimizing unwanted reflections at the surfaces of thin film polarizers. Such coatings are designed to reduce glare and improve light transmission through the material. The application of these coatings can lead to a significant enhancement in the overall efficiency of polarizers.
Anti-reflection coatings create conditions that enhance the usability of polarizers in a broad range of optical applications, from simple projection devices to complex imaging systems.
The composition and thickness of anti-reflection layers are crucial parameters that affect their functionality. By engineering these coatings effectively, it is possible to achieve low reflectance over a wide range of wavelengths, which is a major advantage in many devices. Their application in design architecture reflects the commitment to creating high-performance optical elements.
Manufacturing Techniques for Thin Film Polarizers
The manufacturing techniques for thin film polarizers are central to the understanding of their performance and application. As technology advances, efficient production methods become increasingly crucial for meeting the demands of diverse industries. Thin film polarizers are designed to manipulate light and enhance optical performance. Consequently, knowing how these components are made helps in appreciating their capabilities and implementation in various contexts.
Key elements of manufacturing these polarizers include control over material properties, thickness, and uniformity. Each manufacturing technique contributes uniquely to the successful development of various optical components, sounding the bell for advancements across telecommunications, imaging systems, and display technologies. The following sections detail the primary manufacturing techniques utilized in the industry today.
Physical Vapor Deposition (PVD)
Physical Vapor Deposition, or PVD, is a common technique for creating thin film polarizers. In this method, material is vaporized in a vacuum environment. The vapor then condenses on a substrate, forming a thin layer of the desired film. PVD offers several advantages, including:
- High Uniformity: The process can produce very uniform films, crucial for optimal optical performance.
- Control Over Thickness: Manufacturers can precisely control the film thickness at the nanometer level.
- Wide Range of Materials: PVD can accommodate various materials, enabling customization for specific applications.
However, PVD can be costly and requires advanced equipment, which may limit its use for smaller-scale productions. Additionally, the process can be time-consuming, affecting overall efficiency.
Chemical Vapor Deposition ()
Chemical Vapor Deposition is another prominent method for fabricating thin film polarizers. CVD involves the chemical reaction of gaseous precursors, leading to a thin layer deposited onto a substrate. This method provides several benefits, including:
- Excellent Film Quality: Films produced via CVD typically exhibit superior optical properties.
- Complex Structures: CVD allows for the creation of more complex multilayer structures, enhancing polarization capabilities.
- Versatility: This technique is adaptable for various materials, including organics and inorganics.
Nevertheless, CVD processes can be challenging to optimize. Carefully managing temperature and pressure is vital for ensuring quality deposition. Environmental concerns are also critical, as CVD processes sometimes generate hazardous byproducts.
Sol-Gel Processes
Sol-Gel processes represent an alternative approach for manufacturing thin film polarizers. This technique involves the transition of a solution (sol) into a solid gel phase. The sol is typically a colloidal suspension, containing metal oxides or other precursors. Key benefits of sol-gel processes include:
- Low Processing Temperature: Many sol-gel processes require lower temperatures, facilitating the use of various substrates.
- Flexibility in Composition: This method allows for easy incorporation of different materials, potentially enhancing performance.
- Cost-Effectiveness: Generally, sol-gel processes are more economical compared to PVD and CVD techniques.
Yet, sol-gel methods can lead to various challenges, such as achieving uniform coating thickness and controlling particle size distribution. The drying and curing steps in these processes are also crucial for ensuring the desired properties of the final product.
The choice of manufacturing technique significantly influences the performance characteristics, cost, and effectiveness of thin film polarizers.
Performance Characteristics of Thin Film Polarizers
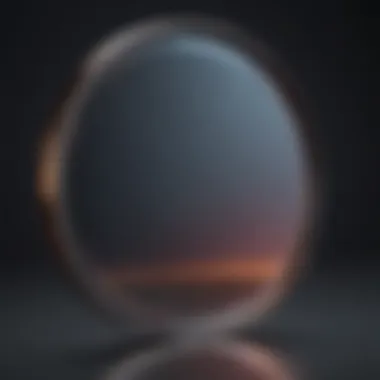
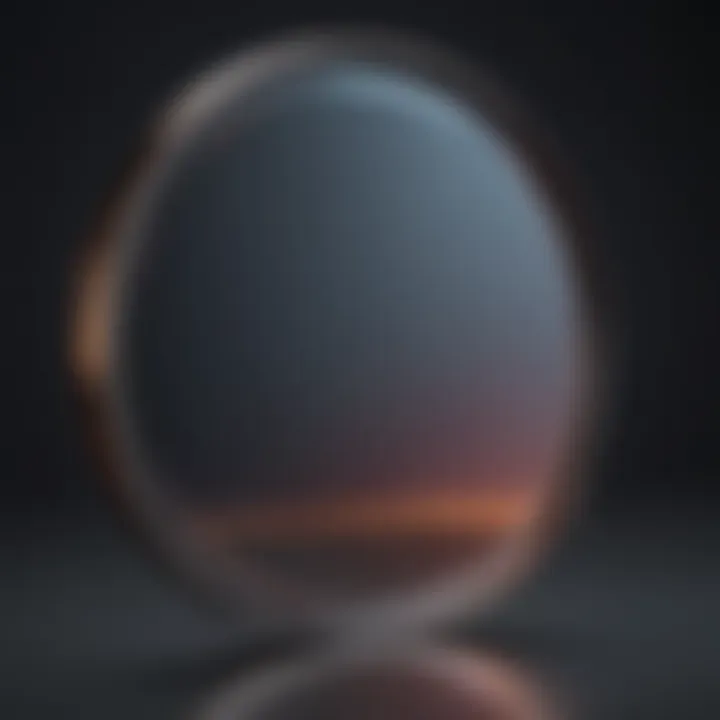
The performance characteristics of thin film polarizers are crucial for their effectiveness in various optical applications. Understanding these characteristics helps in the selection of appropriate polarizers for specific tasks and ensures optimal performance in the desired application. Key elements to consider include the extinction ratio, transmission and reflection rates, and wavelength dependence. Each of these factors plays a significant role in defining how well a thin film polarizer can manipulate light.
Extinction Ratio
The extinction ratio is one of the most important performance characteristics of thin film polarizers. It quantifies the ability of the polarizer to distinguish between polarized light and unpolarized light. The ratio is defined as the ratio of transmitted intensity of light in the desired polarization direction to the transmitted intensity in the orthogonal direction. A higher extinction ratio indicates better performance and efficiency in blocking unwanted light.
Achieving a high extinction ratio requires precise layer design and material selection. Thin film polarizers typically achieve ratios of 100:1 or higher. This characteristic is especially vital in applications such as laser systems and imaging systems, where accurate polarization control is critical. The design might involve multiple layers of dielectric materials to enhance the contrast between the transmitted and reflected light.
Transmission and Reflection
Transmission and reflection rates are pivotal in determining how light interacts with the polarizer. The transmission coefficient indicates how much light passes through the polarizer, while the reflection coefficient measures how much light is reflected back. Ideally, a good thin film polarizer should have high transmission for the desired polarization state and low reflection for the undesired state.
Polarizers are generally designed to optimize these coefficients depending on the intended application. For instance, in optical communication systems, maximizing transmission is crucial to maintain signal integrity. In contrast, imaging systems may prioritize reducing the reflection of stray light to improve image quality. Understanding the specific transmission and reflection performance is key for engineers when designing optical systems that leverage thin film polarizers.
Wavelength Dependence
Wavelength dependence refers to how the performance of thin film polarizers changes with varying wavelengths of light. Typically, thin film polarizers are tuned for specific wavelength ranges. Outside of these ranges, the extinction ratio, transmission, and reflection characteristics may deteriorate.
Designing for wavelength dependence is complex, as it involves a balance between different optical properties. For example, a polarizer may perform excellently at a specific wavelength like 532 nm but may not be effective at longer or shorter wavelengths. This aspect must be considered in applications that span broad wavelength ranges, such as spectroscopy.
The design choices made for thin film polarizers significantly influence their overall effectiveness across different applications.
In summary, the performance characteristics of thin film polarizersβextinction ratio, transmission and reflection rates, and wavelength dependenceβare paramount to their functionality. Understanding these elements equips researchers, students, and professionals with the necessary insights to select and optimize polarizers for various optical applications.
Applications of Thin Film Polarizers
Thin film polarizers are vital components across various optical applications. Their ability to selectively transmit light makes them indispensable in many fields, such as telecommunications, imaging, and display technologies. Understanding these applications helps highlight the growing significance of thin film polarizers in modern technology. They not only enhance performance but also enable innovative solutions to complex optical challenges.
In Optical Communication Systems
Optical communication systems utilize thin film polarizers to improve signal quality. These polarizers filter out unwanted light, allowing only the desired polarization state to pass through. Their role is crucial, particularly in fiber optics, where maintaining signal integrity is paramount. The use of thin film polarizers in these systems can increase the reliability of data transmission over long distances while minimizing interference.
In Imaging Systems
Imaging systems benefit significantly from thin film polarizers. They enhance contrast and reduce glare, which is essential in applications such as photography and microscopy. By selectively filtering polarized light, these polarizers improve image clarity and detail. They also play a crucial role in the design of cameras, where managing reflections can enhance overall image quality.
In Display Technologies
Display technologies have undergone transformative changes thanks to the incorporation of thin film polarizers. These devices ensure light modulation and enhance visual performance in screens.
LCDs
Liquid Crystal Displays (LCDs) use thin film polarizers for contrast enhancement and light manipulation. The key characteristic of LCDs is their ability to control light passage through the liquid crystal layer. This makes them a beneficial choice for displays, as they create vivid images while consuming less power. A unique feature of LCDs is their high color accuracy, which is advantageous in applications demanding precise visual output. However, they can struggle in direct sunlight, leading to visibility issues.
LEDs
Light Emitting Diodes (LEDs) also benefit from thin film polarizers in display setups. Polarizers improve light extraction efficiency, which is a significant aspect in increasing the brightness of LED screens. This characteristic makes LEDs a popular choice for modern displays, contributing to their widespread adoption in various applications. A unique feature of LEDs is their compact size and energy efficiency, which are advantageous in many settings. However, the color dispersion can be a limitation, sometimes requiring additional technology to correct for consistent color representation.
Using thin film polarizers broadens the capabilities and performance of these technologies, playing a crucial role in the advancements of optical systems.
Recent Innovations in Thin Film Polarization Technology
Recent innovations in thin film polarization technology have opened new avenues for research and applications. This area of study focuses on enhancing the performance and functionality of polarizers. Innovations help refine existing technologies while also introducing novel approaches. Understanding these advancements is critical for researchers and professionals looking to push the boundaries of optical sciences.
Nano-Structured Polarizers
Nano-structured polarizers represent a significant advancement in thin film technology. These devices utilize structures on the nanometer scale to control light more effectively than traditional polarizers. This level of precision is paramount for applications that require high efficiency and performance, such as in advanced imaging systems and telecommunications.
The benefits of nano-structured polarizers include:
- Increased Efficiency: They can achieve higher extinction ratios, which means they can significantly reduce unwanted light and improve overall image quality.
- Compact Design: The use of nanostructures allows for thinner and lighter polarizers, which is crucial for modern applications in mobile and portable devices.
- Broader Wavelength Range: These polarizers can be designed to function across a wide range of wavelengths, making them versatile for varied applications.
Research in this area is ongoing, with teams exploring new materials and fabrication processes. They are using advanced techniques such as electron-beam lithography to create these structures with exceptional accuracy. This level of innovation positions nano-structured polarizers as essential tools in both academic research and industry applications.
Active Polarization Techniques
Active polarization techniques represent another frontier in the field of thin film polarizers. These methods enable the dynamic adjustment of polarization states in response to external signals or conditions. As a result, they are suitable for applications where variable light conditions exist.
The core attributes of active polarization techniques include:
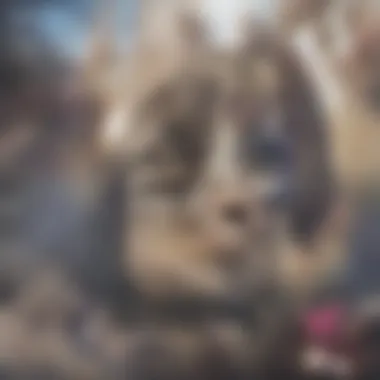
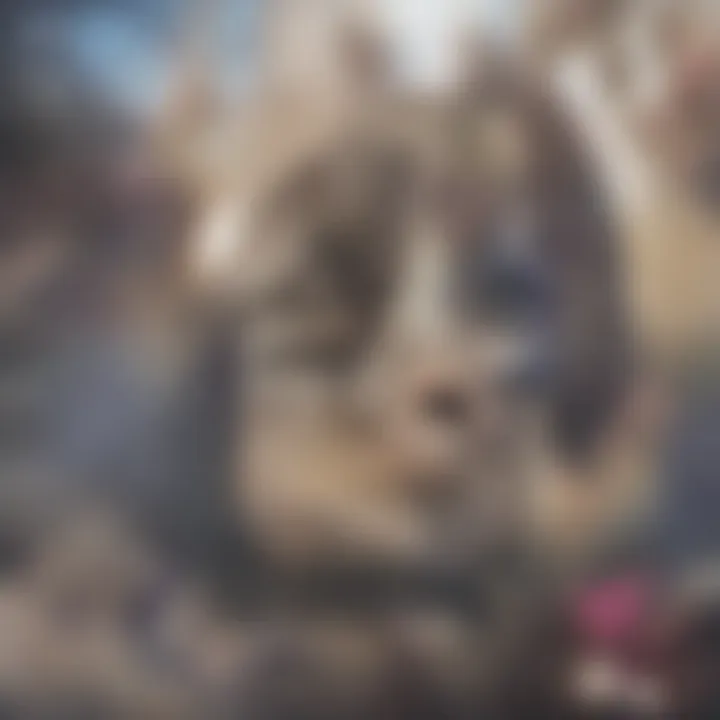
- Real-Time Control: Users can manipulate the polarization state on demand, which is beneficial for adaptive optics and smart optical devices.
- Higher Flexibility in Applications: These polarizers can respond to environmental changes, making them suitable for various fields, including telecommunications, virtual reality, and sensor technology.
- Integration with Electronics: The ability to interface active polarizers with electronic systems enhances functionality, contributing to the development of smarter optical systems.
Despite the advantages, integrating active polarization techniques into existing systems presents challenges. This includes the need for an effective power source, miniaturization of control circuits, and ensuring quick response times. As researchers work through these constraints, the full potential of active polarizers becomes increasingly apparent.
"Advancements in thin film polarization technology underline the industry's commitment to innovation and adaptability."
Challenges and Limitations
The discussion of challenges and limitations pertaining to thin film polarizers is crucial for understanding their practical application and future potential. While advancements in technology continue to enhance performance, certain barriers affect both the materials used and the operational capabilities of these devices. It is vital for both researchers and industry professionals to recognize these constraints to innovate effectively.
Material Limitations
Thin film polarizers typically rely on carefully selected materials that provide the desired optical properties. However, limitations arise based on material performance under different conditions. Common materials like silica, titanium dioxide, and various polymers can exhibit drawbacks such as:
- Temperature Sensitivity: Certain materials may not perform consistently across a wide temperature range. Variations in temperature can affect refractive index and consequently the efficiency of polarization.
- Durability Issues: Some materials are susceptible to degradation over time due to environmental factors. Factors such as humidity and UV exposure can weaken the structural integrity, impacting functionality.
- Cost Constraints: High-quality optical materials can be expensive. This cost can limit accessibility for smaller research initiatives or companies, thus slowing down innovation.
These material limitations can hinder the deployment of thin film polarizers in specific applications where durability and reliability are paramount.
Performance Constraints
In addition to material limitations, thin film polarizers encounter various performance constraints that impact their effectiveness. Some key performance-related issues include:
- Extinction Ratio Limitations: While many thin film polarizers achieve good extinction ratios, the best performance often occurs at specific wavelengths. This can restrict their utility in broader spectral ranges, necessitating specific designs for different applications.
- Angle of Incidence Sensitivity: The performance of thin film polarizers can vary significantly based on the angle of light incidence. This sensitivity can limit their effectiveness in applications where angles cannot be controlled tightly.
- Wavelength Dependence: Certain thin film structures exhibit changes in performance with wavelength variations. This necessitates careful calibration and can complicate their integration into multi-wavelength systems.
Understanding these performance constraints will aid in evaluating thin film polarizers for specific applications, thus enabling advancements that may alleviate some of these challenges.
"Innovation in the field of thin film polarizers must consider both material and performance constraints to advance applications of optical science."
Overall, addressing these challenges and limitations is essential for the future progress of thin film polarization technology. Achieving improvements in these areas can significantly enhance practical applications and expand the horizons for new developments in optics.
Future Directions in Thin Film Polarizer Research
Research into thin film polarizers is evolving. The growing demand for high-performance optical devices drives innovation in this field. Understanding future directions is essential to harnessing the benefits of thin film polarizers in upcoming technologies. This section focuses on two critical elements: integration with new technologies and sustainability considerations.
Integration with New Technologies
Thin film polarizers can significantly benefit from recent advancements in technology. For instance, integration with optical interconnects could enhance communication systems. Polarizers improve signal quality by reducing noise and unwanted reflections. Furthermore, combining them with photonic crystals can result in materials with even higher efficiency.
Another area of integration is into smart coatings. These coatings can adapt based on environmental conditions, leading to potential applications in
- Wearable devices
- Augmented reality
- Autonomous vehicles
Each of these fields presents unique opportunities for thin film polarizers. Their ability to enhance display performance and minimize energy consumption can set the foundation for the next generation of optical systems.
"Integration with emerging technologies will not only enhance the performance of optical devices but also expand their applicability across various sectors."
Sustainability Considerations
As the world becomes more aware of its environmental footprint, sustainability in manufacturing practices cannot be overlooked. Future research in thin film polarizers must consider environmentally friendly materials and processes. This can include the exploration of biodegradable polymers or recyclable materials in the construction of polarizers.
In addition, researchers are looking into minimizing waste during the manufacturing phase. Techniques such as additive manufacturing could provide a solution by reducing material waste. Furthermore, sustainable energy sources should be prioritized in the production of thin films.
The continued advancement in sustainability can lead to benefits such as:
- Lower production costs
- Reduced environmental impact
- Enhanced public perception of optical technologies
Culmination
The conclusion of this article outlines several crucial points about thin film polarizers. Thin film polarizers play a substantial role in various optical applications, enhancing the functionality and efficiency of devices such as cameras, LCDs, and optical communication systems. These polarizers manipulate light effectively, contributing to image quality and information transmission. By examining the core principles, design techniques, and manufacturing processes, professionals can better appreciate the technology's complexity.
Key insights from our exploration are:
- The significance of layer composition in optimizing polarization performance.
- The current limitations that researchers face, which spur ongoing innovation.
- The potential implications of integrating new technologies into polarizer designs.
Summary of Key Insights
Throughout the article, several key insights emerged about thin film polarizers. Firstly, the understanding of light polarization and its applications is essential for advancements in optics. The intricacies of layer structure and the materials used in fabricating these devices contribute to their effectiveness in transmitting and reflecting polarized light. Furthermore, the manufacturing methods such as Physical Vapor Deposition and Chemical Vapor Deposition were explored, highlighting their impact on the quality of thin film polarizers.
A notable point is the impact of recent innovations, particularly with nano-structured polarizers, which expand the scope of polarization technology. The exploration of active polarization techniques also suggests future directions that can be taken in this field.
Implications for Future Research
The future of thin film polarizers is filled with possibilities. Material advancements and the incorporation of sustainability considerations in manufacturing processes are imperative. Future research should also focus on integrating polarizers with emerging technologies like augmented reality, quantum optics, and advanced imaging systems. Such integration can enhance device performance and promote energy efficiency.
Moreover, the ongoing research into active polarizers could lead to adaptive optics applications. These applications may leverage the dynamic control of polarization states, offering enhanced versatility in various optical applications.