Understanding First Energy Wakefield in Particle Physics
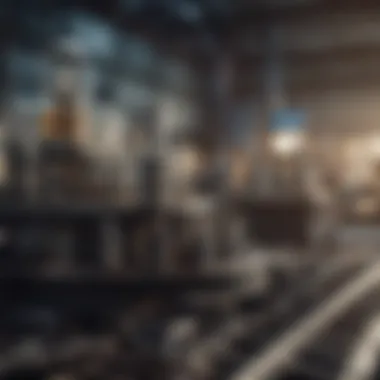
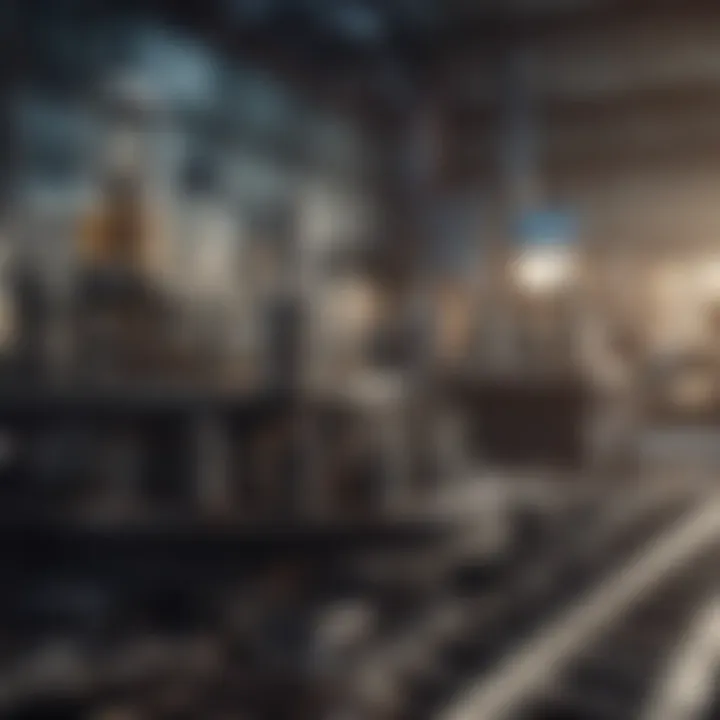
Summary of Objectives
This article investigates the first energy wakefield, a critical phenomenon within particle physics and accelerator technology. The main objective is to outline the principles that guide wakefield generation, detailing the mechanics behind energy transfer and its potential applications in various scientific fields. The exploration aims to elucidate how advancements in this area can impact particle acceleration and the efficiency of accelerator designs.
Importance of the Research
Understanding first energy wakefield can offer significant insights into both theoretical and practical applications. It is important for advancing particle physics research, developing new technologies in accelerators, and fostering innovative approaches in various scientific inquiries.
Preface
The first energy wakefield is increasingly recognized for its pivotal role in enhancing particle dynamics within accelerators. This concept encompasses the intricate behaviors of electromagnetic fields generated by charged particles moving through a medium. The interaction of these fields with the surrounding particles creates a wake, which can be harnessed to accelerate other particles efficiently. This forms the basis for numerous techniques in modern accelerator design.
Results and Discussion
Presentation of Findings
Several key findings arise from ongoing research into first energy wakefield. Experiments demonstrate how varying parameters such as beam intensity and particle charge can significantly alter the characteristics of the generated wakefield. One notable observation is the correlation between wakefield amplitude and the distance of propagation. Increased beam intensity often leads to enhanced field strength, allowing for greater energy transfer to trailing particles.
"The potential for large wakefield amplitudes opens a pathway for compact accelerators to achieve high acceleration gradients."
This understanding makes the concept applicable in diverse areas ranging from medical applications to advanced research facilities. By refining these processes, researchers can enhance the efficiency and precision of particle accelerators.
Implications of Results
The implications of these results are profound. First energy wakefield technology suggests pathways for improving existing accelerators, making them more compact and energy-efficient. The advancements in energy transfer mechanisms could potentially lead to breakthroughs in large-scale collider projects, allowing for better utilization of resources. Additionally, this research aids in understanding fundamental particle interactions at higher energies, paving the way for future explorations in particle physics.
Foreword to First Energy Wakefield
Understanding the first energy wakefield is not merely an academic exercise; it is essential for the advancement of both particle physics and accelerator technology. This section lays the groundwork for comprehending how wakefields operate and their substantial implications for the scientific community. The importance of exploring this concept lies in its capability to lead innovative solutions in particle acceleration techniques, which are critical for current and future experiments in various fields including high energy physics and medical applications.
The concept of first energy wakefield represents a significant breakthrough in energy transfer mechanisms. It has the potential to transform traditional particle accelerators into more compact and efficient systems. As researchers delve into this phenomenon, they uncover opportunities for increased acceleration gradients, which can further enhance the performance and capabilities of these accelerators.
Moreover, this exploration not only emphasizes the theoretical aspects of wakefields but also addresses practical applications that can directly benefit scientists and industry professionals. By understanding these fundamental mechanics, we can envision future advancements that rely heavily on efficient energy transfer at micro and nanoscales.
In summary, examining the first energy wakefield is imperative for anyone invested in the evolution of accelerator technologies and their associated applications in modern science.
Defining Wakefield Phenomena
Wakefield phenomena are established when charged particles pass through a medium, generating electromagnetic fields that influence other particles in their vicinity. Essentially, this process creates waves in the electromagnetic field, which in turn affects the surrounding charged particlesβthese waves are referred to as wakefields. The importance of this definition lies in its broad range of applications, particularly within particle accelerators and laser-plasma interactions.
Wakefields can be classified into two primary types: coherent and incoherent wakefields. Coherent wakefields are generated when a bunch of particles travels in a synchronized manner, while incoherent wakefields arise from random particle motion. Understanding these distinctions offers insights into energy transfer mechanisms and assists researchers in devising methods for optimizing particle acceleration.
History and Evolution of Wakefield Research
The study of wakefield phenomena has a storied history, tracing back several decades. Initial observations began in the mid-20th century as researchers sought to understand how particle interactions could lead to enhanced acceleration techniques. Early experiments primarily focused on identifying the underlying physics of energy transfer and fields produced by charged particles.
As technology progressed, the scope of wakefield research expanded significantly. Notable advancements occurred during the late 20th century, with key experiments that demonstrated the feasibility of using wakefield accelerators as an alternative to conventional methods. This evolution signifies a critical shift in thinking about particle acceleration, pushing the boundaries of what was deemed possible.
Today, ongoing research continues to evolve with various funding initiatives supporting teams worldwide. These efforts aim to harness the full potential of wakefield phenomena, allowing for cutting-edge experimental setups that promise to reshape the landscape of accelerator physics. As scholars continually build upon the accomplishments of their predecessors, the future of wakefield research appears bright, with many new frontiers yet to be explored.
Fundamental Principles of Wakefields
Understanding the fundamental principles of wakefields is crucial in comprehending their role in modern accelerator technology and particle physics. These principles set the stage for exploring how energy is harnessed and transferred between particles via wakefield phenomena. They also offer insights into the design and optimization of compact accelerators that may enhance particle acceleration capabilities.
The Physics Behind Wakefield Generation
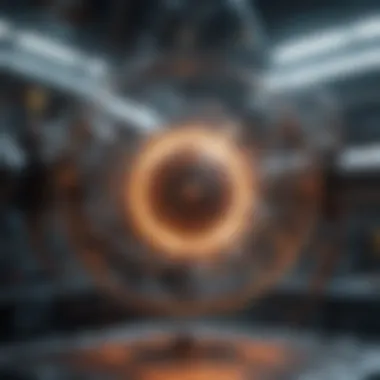
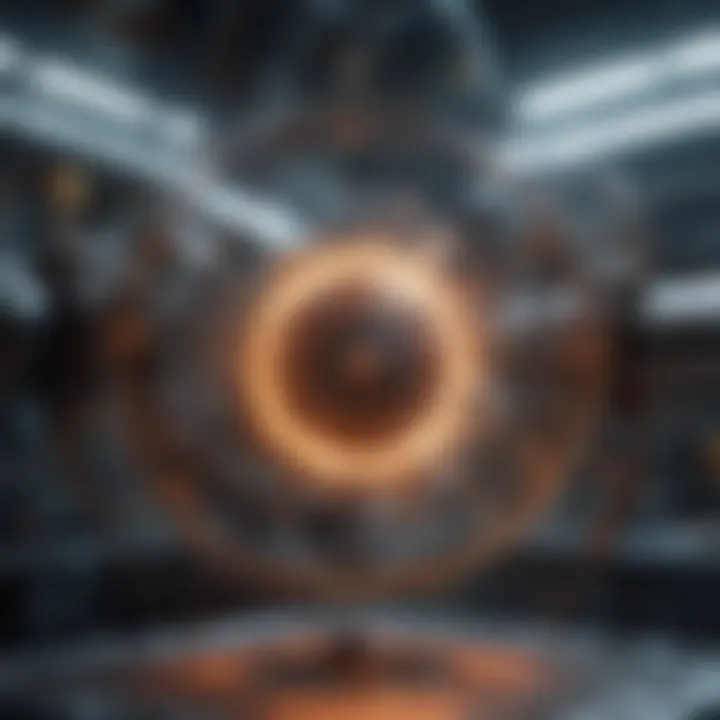
Wakefield generation embodies complex interactions between charged particles and electromagnetic fields. It starts when a charged particle, such as an electron, accelerates through a medium. This acceleration creates disturbance in the surrounding electromagnetic field. The disturbance propagates through the medium, resulting in a wakefield. The essential physics here involves the collective dynamics of the particle motion and the resultant field generation. This phenomenon forms the basis for many applications in particle acceleration, helping in designing particle beam devices that are more effective in energy transfer.
Types of Wakefields
Wakefields can be categorized into different types according to their characteristics and applications. Understanding these types is essential for researchers and engineers working on accelerator design.
Coherent Wakefields
Coherent wakefields arise under conditions where multiple particles contribute synchronously to the electromagnetic field. The key characteristic of coherent wakefields is their ability to generate strong electric fields sufficient for acceleration. This makes coherent wakefields a popular choice for high-performance particle accelerators.
A unique feature of coherent wakefields is that they can produce significant acceleration gradients, enabling a more compact design for particle accelerators. However, they require precise synchronization of particle bunches, presenting a technical challenge for consistent application in experimental setups.
Incoherent Wakefields
Incoherent wakefields, on the other hand, result from random particle motion rather than synchronized behavior. The specific aspect of incoherent wakefields is their contribution to background noise, which may hinder the performance of accelerators.
The key characteristic here is the broad range of frequencies generated, which can be used for various applications beyond simple acceleration. Incoherent wakefields are a beneficial choice when looking to achieve specific measurement techniques or innovative experiments, though they carry the disadvantage of less effective energy transfer compared to coherent wakefields.
Transverse Wakefields
Transverse wakefields are produced by particles moving through a medium in a direction that is not parallel to the primary beam direction. They affect the dynamics of the particles, specifically in terms of lateral displacement. The key characteristic of transverse wakefields is their ability to induce significant steering forces on particles. This property makes transverse wakefields a beneficial choice for applications focused on beam stability and control during acceleration.
A unique feature of transverse wakefields is their potential use in correcting beam instabilities. However, they can introduce complexities in beam dynamics, possibly causing unwanted effects if not managed correctly.
"Understanding the intricate dynamics of different wakefield types is foundational for innovative accelerator designs."
In summation, the fundamental principles of wakefields directly inform the design and application of advanced particle acceleration technologies. Each type of wakefield offers distinct advantages as well as challenges, shaping research directions and experimental methodologies in the field.
Mechanisms of Energy Transfer
Understanding the mechanisms of energy transfer is crucial in the study of first energy wakefield. This section, therefore, serves as an exploration of how energy is transmitted through wakefields and the underlying processes involved.
Energy transfer in the context of wakefield phenomena encompasses various dynamics. It facilitates understanding the conversion of particle energy into electromagnetic fields, which can subsequently invigorate other particles. This dynamic has implications across particle accelerator technologies, especially in designs aiming to enhance efficiency and performance.
A significant aspect of energy transfer involves the interaction between particles and the fields produced in a wakefield. Through this interplay, energy can be harvested and redirected effectively, thus enhancing acceleration processes in particle physics.
- Benefits of Mechanisms of Energy Transfer:
- Improves accelerator design by allowing compact configurations.
- Increases energy efficiency during particle acceleration.
- Supports innovative experiments in fundamental physics research.
In sum, the mechanisms of energy transfer play a pivotal role in realizing the full potential of wakefield technology. It connects theoretical advancements with practical applications, thereby enhancing both our understanding and capabilities in high-energy physics.
Wave-Particle Interaction
Wave-particle interaction is a fundamental concept in the mechanisms of energy transfer within wakefields. It describes how particles, such as electrons, interact with electromagnetic waves generated by the wakefield. This interaction is crucial for energy exchange, leading to various outcomes depending on the conditions.
Several factors influence wave-particle interactions:
- Particle velocity: The speed at which a particle travels relative to the wakefield modifies how energy is transferred.
- Wave properties: Characteristics like amplitude and phase of the wave significantly alter the interaction dynamics.
- Environment: Factors such as plasma density can affect the efficiency of interactions.
This interplay allows particles to extract energy from the wake, accelerating them to higher speeds. Understanding these mechanisms offers insights into both theoretical aspects and applications in particle accelerators.
Field Structures in Wakefields
Field structures within wakefields refer to the arrangement and characteristics of electromagnetic fields produced during wakefield generation. These structures are key in determining how efficiently energy transfer occurs.
The nature of these fields can be complex:
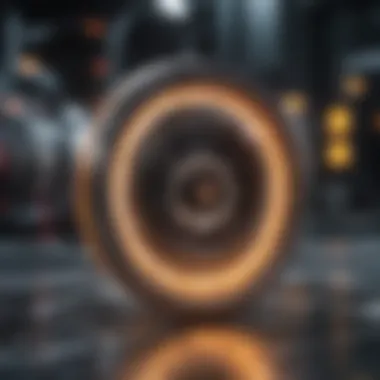
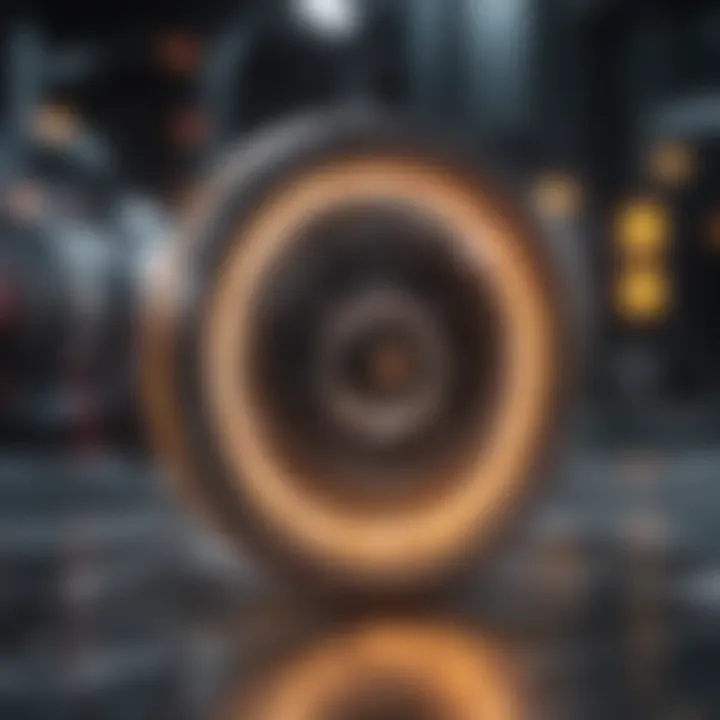
- Types of Field Structures:
- Longitudinal Fields: These are parallel to the direction of beam propagation and help in accelerating particles directly.
- Transverse Fields: These exist perpendicular to the propagation direction and can steer particles, influencing their trajectory.
The efficiency of energy transfer is significantly influenced by the topology of these fields. Effective designs of these structures can maximize particle acceleration and minimize energy losses.
Understanding field structures is essential not just for theoretical research but also for practical applications in designing advanced particle accelerators.
"The design and optimization of field structures in first energy wakefield can lead to groundbreaking advancements in accelerator technology."
Applications of First Energy Wakefield
The Applications of First Energy Wakefield represent a pivotal area in the study of particle dynamics and accelerator technologies. By capitalizing on wakefield phenomena, researchers can harness energy efficiently for various practical applications. This not only enhances the capability of existing technologies but also opens pathways for innovative developments in multiple fields.
Particle Acceleration Mechanisms
Particle acceleration through wakefields is one of the most significant applications. Wakefield accelerators can achieve higher energy levels in shorter distances compared to traditional linear accelerators. They utilize electromagnetic waves generated by a driver, which interacts with particles to accelerate them. This method offers several benefits:
- Compact Design: Wakefield accelerators can be much more compact than conventional systems, leading to reduced infrastructure costs.
- Higher Gradient: They provide greater acceleration gradients, meaning particles can reach high speeds over shorter distances. This is vital for research in high-energy physics where particle collisions are necessary for discovering new phenomena.
- Versatility: They can be adapted for various particle types, including electrons and ions, making them suitable for a broad range of applications.
"The efficiency and compactness of wakefield acceleration make it an attractive option for future particle physics experiments as well as medical applications."
Implications for Laser-Plasma Accelerators
Another domain where first energy wakefields show great promise is in laser-plasma accelerators. These systems use intense laser pulses to create a plasma wave, which then accelerates charged particles. The implications are substantial:
- Energy Efficiency: Laser-plasma systems can produce very high acceleration gradients, significantly improving energy efficiency in particle production.
- Cost-effective Solutions: These accelerators can potentially minimize the costs associated with building large facilities.
- Consideration for Medical Use: They have applications in medical fields, particularly in targeted cancer treatments through proton therapy, which requires high-energy beams in a compact format.
In summary, the applications of first energy wakefield phenomena in both particle acceleration mechanisms and laser-plasma technologies underscore their transformative potential. The ongoing research in this domain highlights the need to advance our understanding of wakefield dynamics further, promising significant strides in scientific investigation and technological innovation.
Recent Advances in Wakefield Research
Recent advances in wakefield research represent a significant leap in our understanding and application of wakefield phenomena. The evolution of this field is crucial for enhancing particle physics and accelerator technologies. Researchers have been exploring innovative methods and technologies that push the boundaries of what was previously possible.
Innovative Experimental Methods
The development of experimental methods has been a cornerstone in wakefield research. A prime focus has been on the use of laser-driven plasma wakefields. These methods allow for the generation of high-quality electron beams with unprecedented energy efficiency. For example, experiments utilizing intense laser pulses to drive wakefields in plasma have shown promising results in accelerating electrons to high energies over short distances.
The integration of diagnostic tools has become ever more essential. Enhanced measurement techniques now allow scientists to track particle dynamics in real-time, offering insights that were previously unattainable. Monitoring wakefield structures through advanced imaging techniques is shedding light on the underlying physics, providing a clearer picture of wave-particle interactions.
Furthermore, collaborative efforts across various research institutions have led to shared knowledge. By combining resources, scientists conduct experiments that span different scales. This multi-faceted approach enriches the experimental landscape and promotes innovative thinking.
Emerging Technologies and Their Impact
Emerging technologies are reshaping the field of wakefield research. One notable development is the use of artificial intelligence in optimizing accelerator performance. AI algorithms can analyze vast amounts of data rapidly, predicting outcomes and helping to refine experimental setups. This has not only sped up the research process but has also increased the accuracy of experimental results.
The development of compact accelerator designs is another striking advancement. With the ability to create high-energy wakefields in smaller facilities, the implications for accessibility and application are profound. Institutions with limited funding now have the potential to conduct high-level research without the need for extensive infrastructure.
Moreover, advancements in materials science have enabled the creation of novel structures that can sustain more robust wakefields. These materials address previous limitations in field strength and energy transfer efficiency, leading to improved designs for future accelerators.
Overall, advancements in innovative experimental methods and technological integration are pushing wakefield research into new territories. As the scientific community continues to unravel the complexities of wakefields, understanding their practical applications remains increasingly important. This field promises to significantly impact future particle acceleration techniques and scientific exploration.
"These recent innovations not only enhance our understanding of wakefield phenomena but also expand the applications of particle accelerators across various fields of research and technology."
As we move forward, acknowledging and fostering these advancements will be crucial for achieving the potential that first energy wakefield research holds for modern science.
Challenges and Limitations
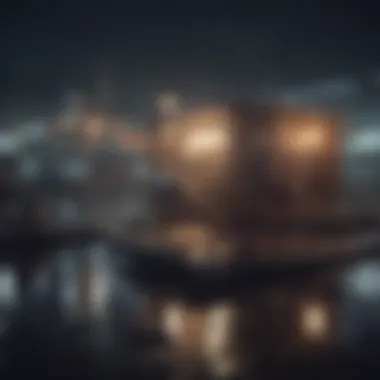
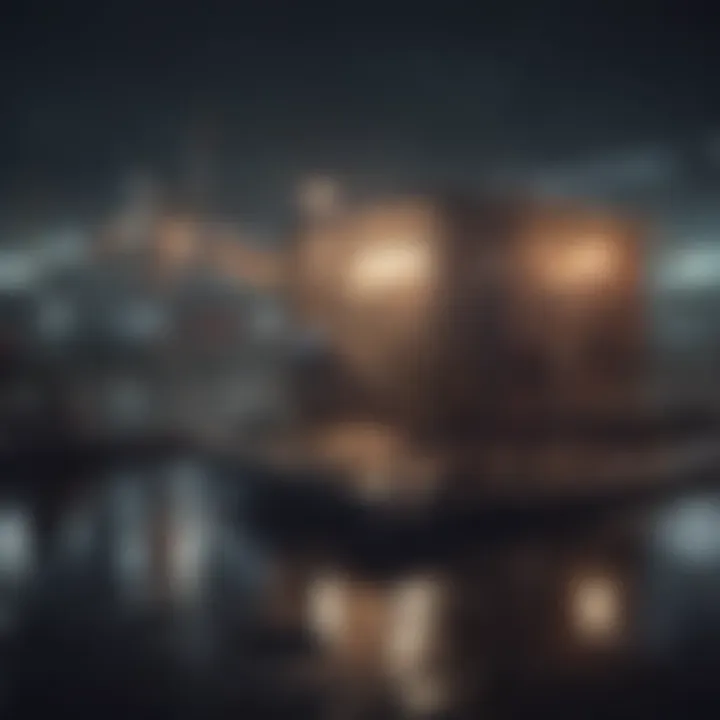
The exploration of first energy wakefield is not without its challenges and limitations. These hurdles can significantly influence the efficiency and applicability of wakefield technology across various scientific disciplines. Understanding these challenges is crucial for researchers and engineers who strive to advance this area of particle physics. In this section, we will examine specific technical constraints and theoretical challenges that impact the field of wakefield generation.
Technical Constraints in Wakefield Generation
Technical constraints are among the most significant challenges encountered in wakefield generation. These limitations stem from the complex nature of the systems involved. For instance, achieving stable and coherent wakefield patterns often requires finely tuned parameters that can be delicate to manage.
Some notable technical constraints include:
- Beam Quality: The quality of the particle beams used can greatly affect the generation of wakefields. Imperfections in beam properties often lead to inconsistent results.
- Energy Efficiency: Many current techniques for generating wakefields are not energy efficient. The high energy demands can deter the feasibility of practical applications in larger scale setups.
- Material Limitations: The choice of materials used in wakefield devices must withstand intense electromagnetic fields. Current materials may not always meet the necessary resistance requirements, causing failure during experimentation.
These technical constraints highlight the need for ongoing research and development in materials science and beam dynamics to improve wakefield generation.
Theoretical Challenges
While technical constraints are concrete obstacles, theoretical challenges present a more abstract but equally vital set of issues. These challenges stem from the current understanding of wakefield physics, which may not fully capture all phenomena involved in high-energy environments.
Important theoretical challenges include:
- Complex Field Dynamics: Understanding the intricate dynamics of wakefields, especially under varying conditions, remains a challenge. This complexity can lead to confusion and misinterpretations in experimental results.
- Model Limitations: Existing theoretical models may not accurately predict wakefield behavior in all scenarios. New approaches are needed to create models that can adapt to a wider range of parameters.
- Interdisciplinary Gaps: Bridging gaps between the disciplines involved in wakefield research, such as plasma physics and accelerator physics, poses challenges in achieving a holistic understanding.
"Overcoming theoretical challenges is essential for transitioning the research insights into practical applications in first energy wakefield technology."
Addressing these theoretical limitations requires input from diverse fields, promoting collaborations among researchers to foster innovative ideas.
Future Directions in First Energy Wakefield Research
The field of first energy wakefield research is on the brink of transformative advancements. Continued exploration in this area holds promise for enhancing the efficiency and scope of particle accelerators. This section will discuss specific elements that can impact future developments and the benefits these innovations may present.
Next-Generation Particle Accelerators
Next-generation particle accelerators are crucial for advancing scientific discovery. With the capacity to produce high-energy collisions, they broaden experimental horizons. First energy wakefields are essential in refining these accelerators. Collaborations among researchers can lead to designs that harness wakefield technology effectively.
These new designs may integrate laser-driven acceleration techniques, allowing for smaller and more cost-effective solutions. The potential to achieve unprecedented energy levels will advance studies in fundamental physics, materials science, and medical applications. The implications might include:
- Increased energy efficiency
- Enhanced particle beam quality
- Reduction in the overall physical footprint of accelerators
Innovative methods can potentially revolutionize how experiments are conducted at facilities like CERN or SLAC, allowing researchers to push the boundaries of current technologies.
"The future of physics may hinge on our ability to effectively utilize wakefields in accelerator designs."
Interdisciplinary Collaborations
Interdisciplinary collaborations are a vital component for the evolution of first energy wakefield research. The integration of knowledge from various scientific disciplines can deepen our understanding of wakefield phenomena. Expertise in fields such as materials science, optics, and quantum mechanics can be invaluable. Researchers need to work across traditional boundaries to innovate faster and overcome challenges that arise in their respective fields.
Some potential benefits of these collaborations may include:
- Development of advanced diagnostic tools for measuring wakefield parameters
- Creation of new materials that can withstand high energy and radiation
- Enhanced computational models for predicting wakefield behavior
An example of successful interdisciplinary work can be found in projects that involve both physicists and engineers. These partnerships can lead to novel techniques and applications, ensuring that first energy wakefield research continues to progress.
End
The conclusion of this article emphasizes the pivotal role of first energy wakefield in the modern landscape of particle physics and accelerator technology. It synthesizes key insights garnered throughout the discussion, highlighting not only the theoretical underpinnings but also the practical applications of wakefields.
Recapitulating Key Insights
- Understanding Wakefield Phenomena: The article elucidates the nature of wakefields and their significance in generating energy transfer through wave-particle interactions. This knowledge is critical for advancing accelerator technologies, particularly in compact designs.
- Mechanisms of Energy Transfer: We examined the specific mechanisms that facilitate energy transfer in first energy wakefields. Recognizing these elements enables researchers to refine existing technologies and develop innovative applications.
- Applications Across Fields: The exploration of potential applications in various scientific realms underscores the versatile nature of first energy wakefields. From particle accelerators to medical technologies, the implications are vast.
- Advancements and Challenges: With ongoing research, significant advancements have been observed, albeit amid various challenges. This duality calls for continued efforts in experimental methodologies and theoretical interpretations.
The Significance of First Energy Wakefield in Modern Science
The significance of first energy wakefield extends beyond theoretical physics. It holds the potential to revolutionize particle accelerators, leading to enhanced performance and energy efficiency. As researchers push the boundaries of what is possible with wakefield technology, several benefits emerge:
- Increased Efficiency: New methodologies promise to maximize energy output while minimizing resource consumption, vital for sustainable scientific progress.
- Innovative Designs: Compact accelerator designs inspired by first energy wakefield principles pave the way for more accessible research facilities across various institutions.
- Collaborative Potential: The interdisciplinary nature of wakefield research invites collaboration, integrating physics with engineering and technology, fostering comprehensive advancements.
- Broader Impact: The implications of these advancements extend into fields like medical imaging, cancer treatment, and materials science, demonstrating that first energy wakefield is not merely a niche topic but a pivotal element in modern scientific endeavors.