Fluorescence Microscope Light Sources Explained
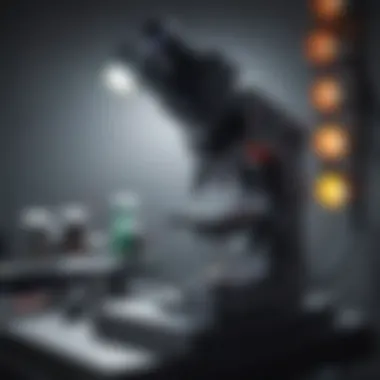
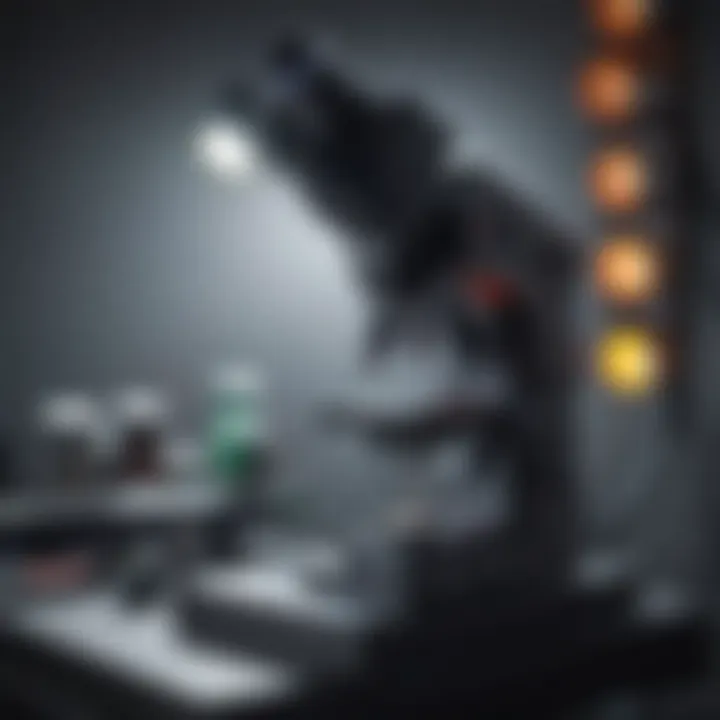
Intro
Fluorescence microscopy has carved its niche in the annals of biological research, allowing scientists to unravel the mysteries lying deep within cellular structures. The role of light sources is not merely an accessory but a cornerstone of this remarkable technique. Understanding how different types of light sources function and their characteristics is fundamental for researchers aiming to achieve optimal imaging outcomes.
Whether you're observing protein interactions, tracking cellular processes, or studying tissue samples, the choice of light source can profoundly affect the clarity, resolution, and depth of the images captured. Each light source spotlights different aspects of the specimens, making it essential to grasp the workings behind them.
Lighting plays a vital role in determining the effectiveness of fluorescence microscopy. The way light interacts with biological samples can lead to different visual narratives. These insights into light sources not only enhance our understanding but also shape experimental designs and the future of research methodologies.
As we navigate through this article, prepare to dive into a detailed exploration of these light sources. We'll highlight their operational principles, advancements in technology, and how all this interconnects with the results observed through the microscope.
Prologue to Fluorescence Microscopy
Fluorescence microscopy represents a cornerstone of modern biological research, providing researchers with the ability to visualize cellular structures and processes in great detail. The technique is imperative for understanding the complexities of life at a microscopic level, giving insights that ordinary lighting methods simply can't provide. With the ability to highlight specific molecules within cells, it offers a fine-tuned look at biological activities, pathologies, and interactions.
Definition and Purpose
At its core, fluorescence microscopy utilizes the properties of fluorescence to enhance the visibility of biological specimens. In simple terms, it involves the excitation of fluorescent dyes or proteins, which absorb light at specific wavelengths and emit light at longer wavelengths. This characteristic allows researchers to distinguish between different cellular components by labeling them with unique fluorescent markers.
The purpose of this technique is manifold: it enables scientists to track biological processes in real time, visualize molecular interactions, and identify the localization of proteins and other molecules within cells. Utilizing fluorescent markers, researchers can paint a picture of cellular environments as they change dynamically over time, thus revealing the hidden corridors of cellular behavior.
"Fluorescence microscopy has surged to the forefront of biomedical research, not just enhancing our visual capacity but expanding our understanding of what lies beneath the cellular surface."
Historical Context
Fluorescence microscopy, while currently part of the standard toolkit for many life scientists, has a rich historical lineage. The fundamental principles date back to the early 19th century when scientists first identified fluorescence as a phenomenon. It wasn't until the mid-20th century that the integration of fluorescence into microscopy really took off, thanks to advancements in both optical technology and camera capabilities.
The invention of the first fluorescence microscope around the 1930s was a game changer. Researchers like Albert Coons pioneered the use of fluorescent dyes to visualize antibodies, laying the groundwork for what would become a standard practice in immunology and cell biology. Over the decades, technological improvements have allowed for greater sensitivity and resolution, hastening the development of fluorescence techniques.
Todayβs systems include sophisticated light sources, advanced cameras, and robust software for image analysis, which simply didn't exist in the earlier days of microscopic research. This evolution not only highlights the ingenuity of modern science but also emphasizes the crucial role that light sources play in achieving the remarkable results seen in fluorescence microscopy.
Mechanics of Fluorescence
Exploring the mechanics of fluorescence is crucial for unpacking the subtleties of fluorescence microscopy. At its core, this method relies on the unique properties of certain molecules that absorb light and re-emit it, allowing researchers to visualize structures that would otherwise remain hidden. Such a process not only enhances our understanding of cellular functions but also has implications across various fields of biological research.
The significance of understanding the underlying mechanics cannot be understated. Knowing how fluorescent molecules interact with light provides insights into how to optimize imaging techniques, improve accuracy in biological interpretations, and ensure reliable results in experiments. Furthermore, a grasp of these principles aids in the selection of appropriate fluorescent markers for specific applications, which enhances both the resolution and quality of images obtained during observations.
Fluorescent Molecules
Fluorescent molecules, or fluorophores, are the stars of fluorescence microscopy. They exhibit the ability to absorb light at one wavelength and emit it at another, longer wavelength. This dual behavior is fundamental for distinguishing between the background signals and the signals of interest in imaging. Typically, certain characteristics define the effectiveness of these molecules. Consider the following key points:
- Brightness and Stability: A fluorophore must be bright enough to stand out against background noise. Stability is also essential; unstable molecules may photobleach quickly, diminishing their utility in prolonged studies.
- Photochemical Properties: The manner in which the molecules respond to excitation light can vary significantly. Some might maintain their ability to fluoresce longer than others, influencing their practicality across different applications.
- Compatibility: The selection of fluorescent markers must fit the light source's spectral output. For instance, if the light source emits primarily blue light, suitable fluorescent molecules should absorb in that range and fluoresce in a detectable range afterward.
All these points highlight the need for careful selection when utilizing fluorescent molecules to achieve the desired outcomes in microscopy.
Excitation and Emission
The principles of excitation and emission are pivotal in the operational framework of fluorescence microscopy. Upon absorption of light, a fluorophore enters an excited state. This is a transient phase, and the excited state cannot persist indefinitely. The fluorophore eventually returns to its ground state, releasing energy in the form of lightβand this is the emission we want to capture.
To delve deeper:
- Excitation: The precise wavelength that triggers the fluorophore's transition to an excited state is known as the excitation wavelength. Selecting the appropriate wavelength is crucial. It must be aligned with the absorption spectrum of the chosen fluorescent molecule. Too long or too short of a wavelength may either fail to excite the molecule or cause it to emit undesirably.
- Emission: When a fluorophore returns to its original state, it emits light at a specific wavelength, usually longer than the excitation wavelength. This characteristic spectral shift is essential for the design of fluorescence microscopy systems, as filters are imposed to separate emitted light from excitation light.
The relationship between the excitation and emission processes ultimately shapes the quality of the images generated. Higher contrasts and better resolutions can be achieved through effective selection and manipulation of these parameters. For example, using filters that perfectly match the emission peak can dramatically enhance image quality.
The excitation and emission characteristics of fluorophores are fundamental to maximizing the effectiveness of fluorescence microscopy.
Light Sources for Fluorescence Microscopy
In the realm of fluorescence microscopy, the light source is the heartbeat of the entire system. It's not just a mere tool; it shapes the way we visualize cellular structures and biological processes, providing insight that significantly aids research in life sciences. The type of light source directly influences the quality and durability of the fluorescent signal, making it crucial to know the ins and outs of each option available. Each light source comes with its unique benefits and drawbacks, impacting aspects like resolution, brightness, and overall imaging capabilities.
Types of Light Sources
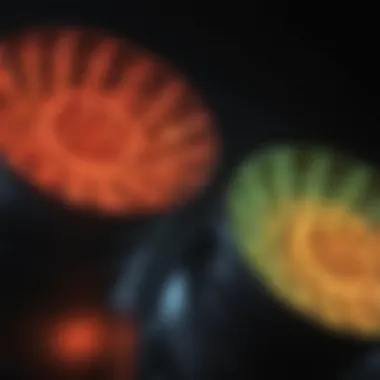
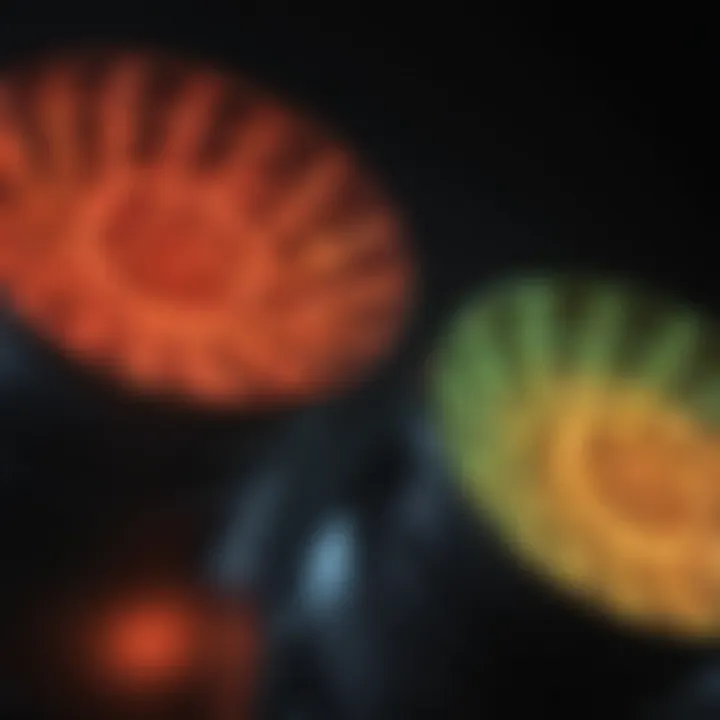
Xenon Lamps
Xenon lamps are one of the traditional stalwarts in the field of fluorescence microscopy. They generate a broad spectrum of light, ranging from ultraviolet to visible wavelengths. This broad output is, in fact, their key characteristic. The benefit here is that they can excite a wide variety of fluorophores effectively, making them an attractive choice for multi-channel imaging.
However, there's a flip side to their brilliance; they can have a shorter life span than other sourcesβoften needing replacement after hundreds of hours of use. Their intensity can also lead to thermal stress on delicate biological samples, which poses a significant challenge in experiments that require prolonged observation.
Mercury Vapor Lamps
Mercury vapor lamps have been the workhorse of fluorescence microscopy for decades. They emit intense bursts of light, particularly in the UV and violet regions, which act as excellent stimulators for many fluorescent dyes. The unique feature of mercury vapor lamps lies in their high intensity, allowing for quick imaging and a wider signal-to-noise ratio, making them a popular choice for applications demanding high sensitivity.
Yet, these lamps come with considerable downsides. They can produce unwanted heat, requiring additional cooling systems to prevent sample damage. Furthermore, the variable output over time can make reproducibility of results a tricky endeavor.Sometimes, you might feel like you're chasing shadows when trying to standardize your imaging conditions.
LEDs
Light Emitting Diodes, or LEDs, have recently taken the spotlight in fluorescence microscopy. Theyβre celebrated for their energy efficiency and long operational life, which can stand up to thousands of hoursβ a stark contrast to other light sources. Their key characteristic is their ability to be easily tuned to specific wavelengths, allowing researchers to selectively stimulate particular fluorophores without fuss.
Despite their advantages, LEDs may not deliver the sheer intensity that other options do, particularly in applications requiring very high-resolution imaging. However, advancements in LED technology have been rapidly bridging these gaps, making them increasingly popular in labs worldwide.
Laser Sources
Laser light sources represent the pinnacle of precision in fluorescence microscopy. They deliver focused beams of light at specific wavelengths, which allows researchers to excite particular fluorophores without affecting others in the vicinity. Their unique feature is their coherence and monochromatic output, which enhances the resolution significantly and is especially suitable for applications like single-molecule imaging.
On the downside, lasers can be quite costly and might require more precise alignment and maintenance. This can lead to complex setups that may be intimidating for new users. However, when used correctly, the advantages they offer often outweigh these drawbacks, making them a staple in advanced fluorescence microscopy applications.
Comparison of Light Sources
Comparative analysis of the light sources can provide deeper insights into the best choices for specific applications. Let's summarize key elements:
- Intensity: Mercury vapor lamps and lasers offer high-intensity output; Xenon lamps follow closely.
- Lifetime: LEDs stand out with their longevity, while Xenon and mercury lamps have shorter operational lives.
- Flexibility: Xenon lamps and LEDs can accommodate various fluorophores, whereas lasers are more specialized.
Choice of light source significantly influences the imaging performance; understanding your specific needs shapes better research outcomes.
Operational Principles of Light Sources
Understanding the operational principles behind light sources is crucial for anyone delving into fluorescence microscopy. This knowledge not only enhances the imaging quality but also plays a critical role in selecting the right light source for specific applications. These principles encompass how light interacts with fluorescent samples, as well as the characteristics of the light being used. It's a dense topic, yet essential for achieving optimal results in biological imaging.
Spectral Output and Wavelength Selection
The spectral output of a light source refers to the range of wavelengths it emits. Different fluorescent molecules absorb and emit light at specific wavelengths. Understanding this relationship is vital in selecting suitable filters and light sources. For instance, if you are working with a fluorescent dye that peaks at 488 nm, using a light source that effectively emits in that range ensures maximum excitation. The emitted light must also meet the detectors' requirements for capturing clear images.
Here are some points worth considering:
- Fluorophore Selection: Different fluorophores respond to different wavelengths, making the compatibility of your light source and fluorophore a priority.
- Filtering: The right filters must be employed to eliminate unwanted wavelengths and ensure only the selected excitation wavelength reaches the sample.
- Multiple Fluorophores: When your project involves multiple fluorophores, spectral output becomes particularly complex. Youβll need a light source capable of exciting all the chosen dyes without significant spectral overlap.
This selection process is akin to picking the right tool for a job; using a mismatched tool, or in this case, a light source, could lead to poor results.
Intensity and Stability
Intensity and stability are other crucial elements. In fluorescence microscopy, the emitted signal must be strong enough to be distinguished from the background noise. A bright light source can significantly enhance image clarity by providing a robust signal from the fluorescing molecules. However, this intensity doesnβt matter much if it isnβt stable; fluctuations in light intensity can lead to inconsistent results, affecting the reliability of images.
- Intensity Calibration: Regular calibration of the light source ensures youβre working with accurate intensity levels. This step can help mitigate issues arising from gradual dimming or increases in brightness.
- Thermal Stability: The operating temperature impacts stability. Overheating can alter the spectral properties or reduce the lifetime of certain light sources, particularly LEDs and lasers.
- Uniformity of Output: A consistent output across the field of view helps avoid artifacts in images. Non-uniform light can lead to areas of overexposure and others that appear dim, complicating analysis.
Acquiring a light source that balances both intensity and stability is akin to walking a tightrope. Too much intensity without stability, or stability without intensity, can misguide the scientific narrative a researcher aims to present.
"The quality of fluorescence microscopy is directly tied to the careful selection and understanding of light sources, making operational principles a foundational component of the technique."
In summary, grasping these operational principles sheds light on not just how fluorescence microscopy works, but why precise selection and management of light sources can profoundly affect the outcomes observed in your imaging pursuits.
Advancements in Light Source Technology
The field of fluorescence microscopy has witnessed notable progress over the years, primarily due to advancements in light source technology. Light sources are more than just tools; they are the heart of fluorescence microscopy techniques, guiding researchers toward deeper insights into cellular structures and biological processes. Therefore, understanding the nuances of these advancements can enhance imaging quality significantly.
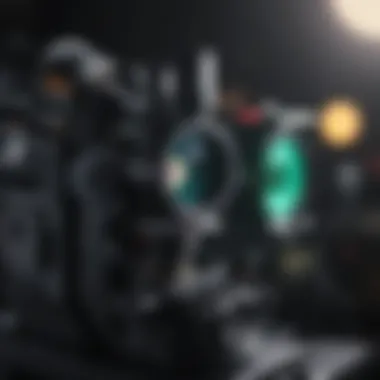
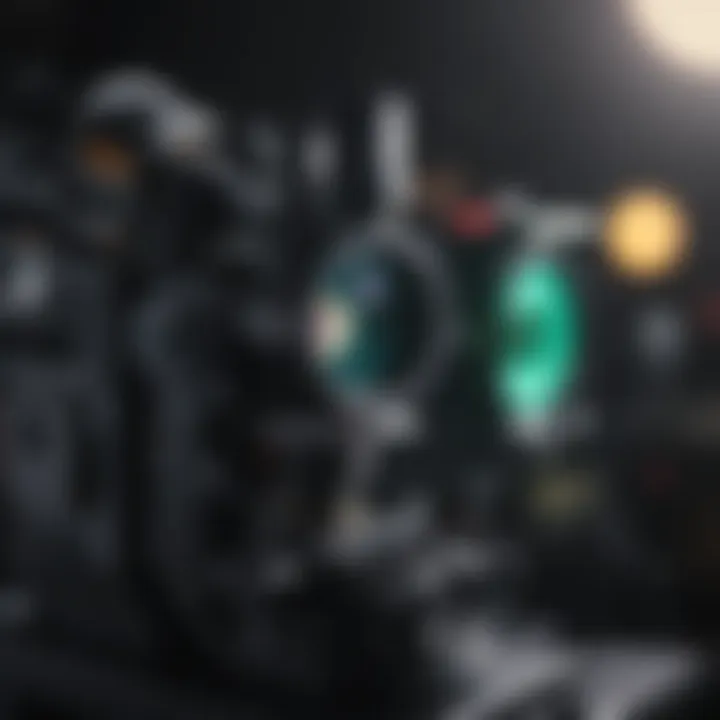
With the emergence of new technologies, researchers now have access to light sources that not only improve imaging fidelity but also increase the overall efficiency of experiments. Each advancement brings with it specific benefits, such as reduced phototoxicity, enhanced stability, and broader spectral ranges, which are critical for multi-channel imaging. By adopting these modern innovations, scientists can extract more detailed information from their samples, making discoveries that were previously impossible.
Solid-State Lasers
Solid-state lasers represent one of the most transformative developments in fluorescence microscopy. Unlike traditional sources, these lasers emit a narrow wavelength range of light, allowing researchers to target specific fluorescent molecules with remarkable precision. They are known for their exceptional stability and high intensity, which translates to improved signal strength.
One significant advantage of solid-state lasers is their long lifespan and low maintenance requirements compared to other light sources like mercury vapor lamps. Plus, they generate less heat, reducing the risk of thermal damage to sensitive samples and minimizing photobleaching. This feature is especially beneficial when observing live specimens, as it allows for longer observation times without compromising integrity.
Moreover, solid-state lasers can be tuned to various wavelengths, facilitating multi-color imaging without needing multiple light sources. This flexibility opens the door for more complex experiments where researchers study different cellular components simultaneously, yielding rich data sets for analysis.
Advancements in LED Technology
Light Emitting Diodes (LEDs) have revolutionized the fluorescence imaging landscape, providing a viable alternative to traditional lamp sources. The advantages of LED technology in microscopy are too compelling to overlook. First and foremost, LEDs offer remarkable energy efficiency, providing bright illumination while consuming far less power than their predecessors.
LEDs are known for their longevityβoften lasting thousands of hoursβwhich sharply reduces replacement costs. This long life, alongside minimal heat production, ensures samples remain unharmed, which is particularly crucial in sensitive applications like live-cell imaging.
Additionally, the spectral range of LEDs has improved significantly. Modern LED systems can cover a range of wavelengths that cater to various fluorophores, allowing for a wide array of experiments without the need for numerous, cumbersome light sources. In practices, such flexibility means that researchers can quickly switch between different fluorescent tags, enhancing throughput in high-throughput screening assays.
In summary, the advancements in light source technology, particularly through solid-state lasers and LED innovations, have not merely enhanced fluorescence microscopyβthey have fundamentally changed how scientific inquiry is approached in the life sciences. As researchers continue to explore the intricacies of biological systems, these cutting-edge light sources promise to deliver even more exciting opportunities for discovery.
Impact of Light Source Characteristics on Imaging
The characteristics of light sources play a pivotal role in the outcomes of fluorescence microscopy. Understanding how these attributes affect imaging can significantly enhance experimental results. Researchers must pay close attention to several key elements, such as the spectral output (wavelength), intensity, and stability of the light sources they choose. Each of these factors contributes to the clarity and accuracy of the images obtained, which is crucial for drawing reliable conclusions in biological research.
Resolution and Contrast
Resolution in fluorescence microscopy refers to the ability to distinguish between two closely spaced objects. The challenge often lies not just in the optics but fundamentally in the light source itself. Light sources with narrow spectral bands can significantly improve resolution, enabling the detection of fine structures within cells. For instance, a laser source that excites a specific fluorochrome can minimize background emissions, thereby enhancing the visibility of the target structures.
Conversely, contrast is vital for differentiating between varying components within a cell. A light source's intensity directly impacts contrast levels. When the excitation light is too dim, it may fail to excite sufficient fluorophores, leading to poor contrast; on the other hand, excessively intense light can saturate the detector, washing out fine details. Consequently, a balance must be struck. If a source like an LED provides stable and tunable intensity, it can maintain optimal contrast without compromising resolution.
"The combination of high resolution and strong contrast is the hallmark of successful fluorescence imaging, enabling researchers to decipher intricate biological processes."
In practical applications, itβs not uncommon to see different light sources being employed in tandem to optimize both resolution and contrast. For example, in studies involving proteins expressed in living cells, a combination of laser scanning confocal and wide-field fluorescence microscopy may be used, each contributing its unique advantages to reveal detailed cellular phenomena.
Signal-to-Noise Ratio
Signal-to-noise ratio (SNR) is another critical factor when evaluating light sources in fluorescence microscopy. Simply put, SNR measures the strength of the desired signal against the background noise present in the system. High SNR indicates clearer images, whereas low SNR obscures details, making it difficult to analyze biological specimens effectively.
Several aspects of light sources influence the SNR. For instance, a mercury vapor lamp might provide strong excitation light, but if its spectral profile overlaps significantly with emission wavelengths, it can lead to increased background noise. In contrast, solid-state lasers can be engineered to have a spectral purity that minimizes this overlap, thereby enhancing the SNR.
Moreover, imaging techniques like time-gated detection can further improve SNR by only recording signals at specific times when the desired emissions occur, filtering out extraneous signals generated by ambient or incidental light.
The challenge for researchers is to select a light source that not only excites the fluorophores effectively but also delivers a high SNR over the relevant imaging area.
In summary, the impact of light source characteristics on imaging in fluorescence microscopy cannot be overstated. From resolution and contrast to signal-to-noise ratio, the ability to manipulate and optimize these elements is crucial for success in biological research. As the techniques and technologies evolve, the understanding of these principles continues to deepen, urging a considered approach to light source selection in microscopy.
Considerations for Optimal Imaging
When engaging with fluorescence microscopy, the right light source isnβt merely a detail; it fundamentally shapes the imaging process. Choosing an optimal light source hinges on a variety of factors that can influence the clarity, resolution, and overall success of an imaging experiment. Understanding these considerations is crucial for producing reliable, interpretable results, especially in complex biological studies.
Choosing the Right Light Source
Selecting the appropriate light source involves considering the nature of the specimens being studied and the anticipated outcomes. Not every light will illuminate your target equally well. \n Here are key factors to think about while making this decision:
- Wavelength Compatibility: Different fluorophores are sensitive to specific excitation wavelengths. For instance, if one is examining GFP-labeled proteins, using light in the blue spectrum is essential to excite the fluorophore effectively.
- Intensity and Stability: A consistent and stable intensity ensures that images are not marred by fluctuations that can obscure details. For longitudinal studies, stability is key to ensuring that the imaging conditions remain unchanged over time.
- Heat Management: Excessive heat from certain sources can damage sensitive samples. Thus, itβs wise to look into light sources that maintain a lower thermal output, especially when working with live cells.
- Cost and Availability: Different light sources come with varying costs and accessibility. Researchers need to factor in their budgets and availability of each light type in their respective laboratories.
Using the wrong source could lead to poor excitation of the fluorescent markers or undesirable photodamage to the specimens, which is why thorough consideration is vital.
Managing Photobleaching
Photobleaching refers to the loss of fluorescence of a specimen due to prolonged exposure to light. This phenomenon can significantly compromise the integrity of imaging, especially in experiments that require observing dynamic biological processes over time. Implementing effective strategies to mitigate this is important for good microscopy practice.
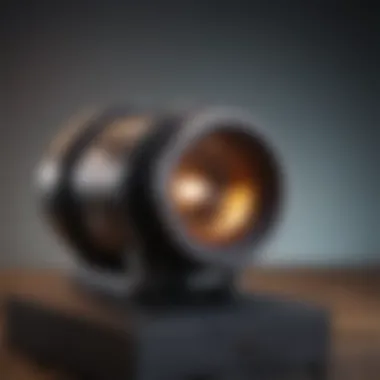
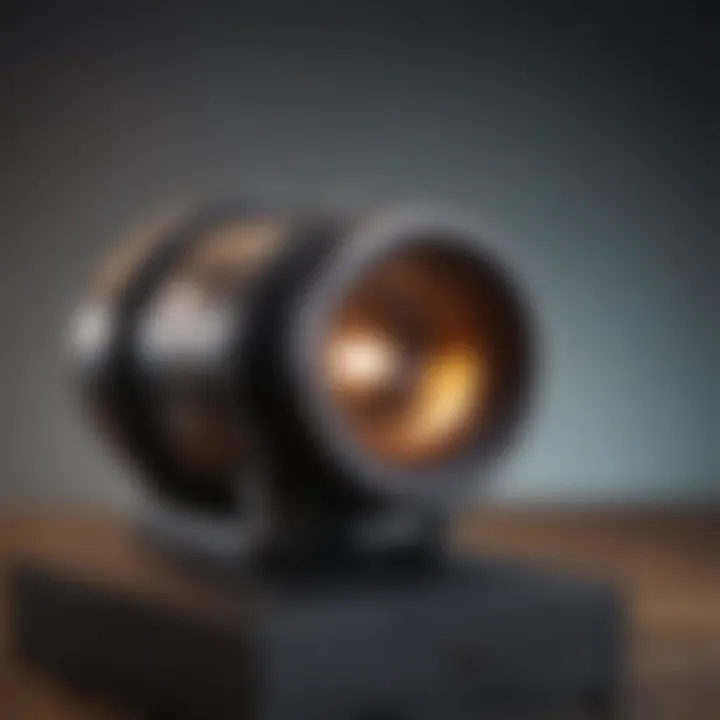
To manage photobleaching effectively, consider the following:
- Minimize Exposure Time: Whenever possible, reduce the duration that the specimen is exposed to excitation light. Implementing fast imaging techniques can help capture data with minimal photodamage.
- Use of Antifade Reagents: These compounds can inhibit photobleaching, allowing the specimens to retain their fluorescence properties longer. Such reagents are indispensable when conducting extensive imaging sessions.
- Adjust Light Intensity: Sometimes, less really is more. Lowering the intensity of the excitation light can help reduce the rate of bleach without overly sacrificing image quality.
- Select Appropriate Filters: Utilizing filters designed to block unnecessary wavelengths can minimize unwanted exposure and protect your specimen from unnecessary light.
By balancing appropriate light source selection with careful control of light exposure, researchers can significantly enhance both the quality of their images and the lifespan of their fluorophores.
"The key to successful fluorescence microscopy often lies in the detailsβattention to light source and photobleaching can redefine what is possible in imaging."
Applications of Fluorescence Microscopy
Fluorescence microscopy stands as a cornerstone in the toolkit of modern biological research. Its capacity to reveal intricate details of cellular components comes from the use of fluorescent dyes and tags that illuminate specific molecules, thus providing insights that traditional microscopy may miss. The implications of these applications stretch far beyond mere visualization; they forge connections between biochemical processes and cell function, driving innovations across various fields.
Fluorescence microscopy finds utility in diverse settings, but two vital areas stand out: cell biology and virology/pathology. Each of these fields harnesses the unique capabilities of fluorescence to delve deeper into the complexities of life at a molecular level. This exploration not only furthers our understanding but also opens the door for novel therapeutic approaches.
"In the realm of cellular investigation, fluorescence is akin to turning on a light in a dark room; it reveals the unseen."
Cell Biology
Cell biology, the study of the structure, function, and behavior of cells, heavily incorporates fluorescence microscopy to dissect cellular architecture and dynamics. With fluorescent markers, researchers can track the localization and movement of proteins within live cells. This capability is crucial for understanding fundamental processes such as cell division and intracellular signaling.
- Visualization of Cellular Components:
Fluorescence microscopy allows for tagging specific organelles, such as mitochondria or lysosomes, enabling precise observation of their functionalities. - Real-time Imaging:
This technique facilitates real-time monitoring of cellular events, as seen in studies where scientists observe the migration of immune cells toward infection sites. - Quantitative Analysis:
Advanced image analysis software can convert fluorescence signals into quantifiable data, leading to insights into protein expression levels or cellular responses.
Through methods such as fluorescence resonance energy transfer (FRET), researchers can even probe interactions between proteins, providing a dynamic view of cellular processes that would otherwise remain hidden. The relevance can't be understated here; it pushes forward not just our understanding of biology but also the development of strategies to combat diseases at the cellular level.
Virology and Pathology
In virology and pathology, fluorescence microscopy is indispensable for diagnosing and studying infectious diseases. The ability to visualize viruses within host cells presents a transformative insight into their life cycles and interactions.
- Detection of Viral Pathogens:
Fluorescently labeled antibodies can target viral proteins, allowing for quick identification of pathogens directly in infected tissues. This specificity enhances diagnostic accuracy and speed. - Understanding Host-Virus Interactions:
By studying the entry, replication, and spread of viruses within cells, researchers can develop targeted antiviral therapies. Visualizing these processes in real-time sheds light on their mechanisms and evolutionary adaptability. - Pathogen Behavior in Tissues:
Fluorescence microscopy enables researchers to observe how pathogens navigate within a host organism, effectively mapping the progression of a disease within biological systems.
These applications not only advance our basic understanding of virology and pathology but have practical implications in developing vaccines and therapeutic interventions.
In summary, the applications of fluorescence microscopy extend far and wide, significantly impacting both cell biology and virology/pathology. By capitalizing on the power of fluorescence, researchers can illuminate the dark corners of cellular biology, paving the way for groundbreaking discoveries and advancements. This synergy between technology and biological discovery exemplifies the importance of continued research and innovation in microscopy.
Future Directions in Light Source Development
Exploring the future of light sources in fluorescence microscopy opens new avenues for enhancing imaging capabilities. This exploration is pivotal for researchers aiming for precision and clarity in visualizing biological specimens. Understanding innovations not only reveals the potential of light sources but also sheds light on their significant role in enabling breakthroughs in life sciences. Advances in technology promise greater sensitivity, higher resolution, and more sophisticated imaging techniques that can be pivotal in unraveling complexities of cellular structures.
Integration with Other Imaging Modalities
One of the exciting future directions in light source development is the integration of fluorescence microscopy with other imaging modalities. Combining techniques, such as confocal microscopy, electron microscopy, or super-resolution microscopy, can create a synergy that leads to richer data sets and more comprehensive analyses.
- Enhanced Data Interpretation: By fusing information from various sources, researchers can correlate molecular signals to precise structural features observed through different imaging methods.
- Improved Sensitivity: The strengths of one modality can offset the limitations of another. For instance, integrating fluorescence microscopy with electron microscopy allows for enhanced contrast and resolution, capturing minute details that fluorescence alone may miss.
- Broader Range of Applications: This integration becomes especially relevant in complex biological systems, allowing for real-time monitoring of cellular dynamics with greater accuracy. In virology research, the interplay of light and electron microscopy could yield insights into viral entry and replication dynamics.
Such integrative approaches require careful consideration of light source compatibility. The spectral outputs must align adequately across the respective modalities, ensuring that the intricacies of the biological samples are effectively illuminated.
Innovations in Light Source Efficiency
As the field moves forward, innovations in light source efficiency become vital. This focus on enhancing efficiency is not merely a matter of improving light output but also involves refining how light is harnessed to optimize its utility in imaging biological samples.
- Solid-State Technologies: The transition to solid-state light sources, like LEDs, promises a multitude of benefits. These light sources consume less energy, produce less heat, and offer longer lifespans compared to traditional mercury or xenon lamps. Such characteristics lead to more sustainable practices in laboratories, minimizing operational costs over time.
- Tunable Sources: Future innovations are likely to include more tunable light sources, allowing researchers to adjust wavelengths on-the-fly. This flexibility can enable targeted excitation of a wider variety of fluorescent probes, thereby enhancing multiplexing capabilities in experiments.
- Minimized Photobleacing: Efficient light sources are also essential in addressing the challenge of photobleaching. By maximizing the effectiveness of light used during imaging, the potential for damaging fluorescent markers is reduced, leading to longer-lasting signals and comprehensive visualizations.
In sum, light sources in fluorescence microscopy are on the brink of revolutionary enhancements. By integrating disparate imaging modalities and improving light source efficiency, researchers can expect continuous advances that not only refine imaging quality but also deepen our understanding of biological systems.
Epilogue
The conclusion serves as a key wrap-up to the extensive exploration of light sources in fluorescence microscopy. It's not merely a summary, but a crucial acknowledgment of how these elements underpin the accuracy and effectiveness of microscopic imaging. Understanding the subtleties of various light sources leads to improved research methods and ultimately enhances our grasp of biological processes at the cellular level.
Summary of Key Points
- Role of Light Sources: The type of light source used dramatically influences image quality, including resolution and contrast. Different sources like Xenon lamps and LEDs have distinct properties that should be selected based on the intended application.
- Operational Principles: The understanding of spectral output and intensity is vital. Light sources should provide stable illumination over appropriate wavelengths to reduce signal variability.
- Technological Advancements: Recent innovations have brought about solid-state lasers and advanced LED technologies which significantly enhance efficiency and reduce costs. Their incorporation into microscopy techniques is revolutionizing how biological samples are analyzed.
- Application Implications: The implications of choosing the right light source extend beyond imaging. It facilitates deeper insights into fields like cell biology and pathology, where precise visualization is paramount.
The Importance of Continued Research
Ongoing research in light source development is essential for several reasons:
- Innovation: Advancements in technology can lead to more efficient imaging modalities. This paves the way for novel applications in diverse fields, extending the boundaries of what can be examined under a microscope.
- Enhanced Understanding: As researchers uncover more about fluorescent proteins and their interactions with light, there emerges a vast potential for new discoveries in cellular dynamics.
- Collaboration with Other Modalities: Future breakthroughs may lie in the integration of fluorescence microscopy with other imaging techniques, leading to a more comprehensive view of biological specimens.
Ultimately, the journey into the depths of fluorescence microscopy emphasizes the importance of light sourcesβthese unsung heroes of biological research. Their evolving nature signals a promising horizon in the quest to unscrew the mysteries held within living organisms.