Genetools: Innovations in Genetic Research and Technology
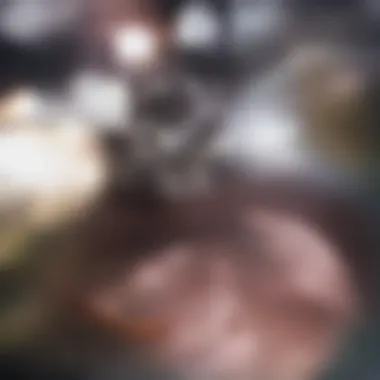
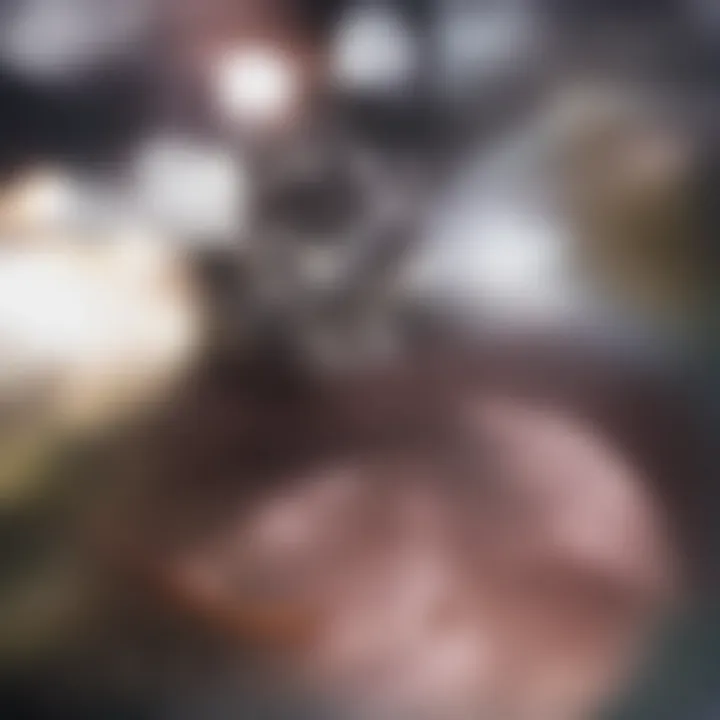
Intro
The field of genetics has rapidly evolved, introducing a variety of powerful tools that reshape our understanding of biological processes. Two prominent examples are CRISPR technology and gene sequencing methods. Their application spans multiple domains including medicine, agriculture, and environmental science. By examining these tools, researchers gain insights that could unlock further advancements in genetic manipulation and understanding.
This article provides a detailed exploration of genetic tools, emphasizing their innovative aspects and implications. Understanding these advances is crucial as they promise significant transformations in how we approach genetic research and its societal impacts.
Foreword to Genetic Tools
Genetic tools play crucial role in modern science. They enable researchers to explore genetic material and understand its implications in various fields, from medicine to environmental science. The use of genetic tools enhances our capacity to manipulate and analyze genes. This leads to breakthroughs in disease treatment, agricultural advancements and improved conservation strategies.
Developing genetic tools requires a blend of technology and biology. Such tools facilitate precision in studies by allowing scientists to perform detailed genetic manipulations. The innovations within genetic research not only provide novel techniques but also raise important questions around ethics and accessibility.
Defining Genetools
Genetools refers to the array of technologies and methods used in genetic research. This includes approaches like CRISPR technology, gene sequencing, and various analysis techniques. Each tool comes with specific features that cater to distinct aspects of genetic research. For example, CRISPR enables targeted gene editing with high accuracy. Similarly, gene sequencing technologies allow for the detailed mapping of genomes, enlightening us about genetic variations and their impacts.
These tools contribute significantly to biotechnology, synthetic biology, and genomics. They form the backbone of innovative research in genetics. The development and refinement of these tools have been ever-evolving. The focus is not only on improving outcomes in lab experiments but also on enhancing applications in real-world scenarios.
Historical Context of Genetic Research
The history of genetic research is rich and complex. Early discoveries laid the groundwork for modern genetic tools. Gregor Mendel's observation of inheritance patterns in the 1860s initiated scientific inquiry into genetics. This was a pivotal moment that led others to explore how traits are passed through generations.
The discovery of the structure of DNA by James Watson and Francis Crick in 1953 marked another crucial milestone. This understanding revolutionized the field, as it revealed the molecular basis for heredity. The emergence of various technologies in the late 20th century, including polymerase chain reaction (PCR), allowed for amplified DNA samples, which opened new doors for research and diagnostics.
As technology advanced, so did the possibilities of genetic research. The 21st century introduced techniques like next-generation sequencing. This technology made it possible to sequence entire genomes quickly and at a low cost. Frame shifts in both ethics and uses of these technologies created a landscape filled with opportunities and challenges that continue to evolve. With these advancements, the ethical considerations surrounding their usage have also gained importance, compelling researchers to navigate this delicate balance.
Classification of Genetic Tools
The classification of genetic tools is essential in understanding their diverse applications and benefits in various scientific disciplines. By categorizing these tools, researchers can more effectively choose the right methods for their specific needs. A well-defined classification promotes clarity and strengthens the foundation for innovations in genetic research. The three major categories in this context include molecular techniques, bioinformatics tools, and laboratory equipment.
Molecular Techniques
Molecular techniques form the core of genetic analysis. They are instrumental in manipulating and understanding the genetic material of organisms. The most significant methods include:
- Polymerase Chain Reaction (PCR): This technique amplifies DNA, allowing scientists to generate millions of copies of a particular DNA sequence. It is crucial for everything from diagnostics to genetic research.
- Gel Electrophoresis: This method separates DNA fragments based on their size. It is often used to analyze PCR products, ensuring that the correct fragments are obtained for further analysis.
- Restriction Enzyme Digestion: This involves cutting DNA at specific sequences, enabling gene cloning and analysis. It helps in constructing recombinant DNA for various applications.
These molecular techniques not only facilitate basic research in genetics but also open doors for their application in medicine, such as in genetic testing and personalized medicine.
Bioinformatics Tools
Bioinformatics tools are vital in managing and interpreting the vast amounts of data generated by genetic research. With the advent of high-throughput technologies, an efficient means to analyze large data sets became essential. Key bioinformatics tools include:
- Genome Assemblers: Software such as SPAdes and Canu that piece together sequences from fragmented data.
- Gene Prediction Tools: Tools like AUGUSTUS predict the locations of genes in a genomic sequence, which is crucial for understanding gene function.
- Databases: Repositories such as GenBank and the Ensembl database store massive amounts of genetic information. Researchers access these resources to gather data for their studies.
The significance of these tools lies in their ability to extract meaningful information from complex genetic data. They enable researchers to make connections that might not be immediately obvious from the raw data.
Laboratory Equipment
Laboratory equipment plays a crucial role in the practical application of genetic tools. High-quality equipment is needed to carry out experiments accurately and efficiently. Key types of laboratory equipment include:
- Thermal Cyclers: Used for PCR, these machines control temperature changes necessary for DNA amplification.
- Spectrophotometers: Instruments that measure the concentration and purity of nucleic acids and proteins, ensuring high-quality samples for further experimentation.
- Automated Pipettes: They enhance precision in sample handling, reducing human error in experiments.
The choice of laboratory equipment influences the efficacy of genetic studies. Proper instrumentation ensures reliable data and contributes to the advancement of genetic research.
The classification of genetic tools is not just for convenience. It shapes the landscape of genetic research and enhances the potential for innovation.
Understanding various types of genetic tools can empower researchers and professionals, leading to informed decisions in their work and contributing to the overall advancement of genetic science.
CRISPR Technology
CRISPR technology stands at the forefront of genetic research, fundamentally altering how scientists approach genetic alteration and experimentation. Characterized by its simplicity, efficiency, and precision, this technology has garnered significant interest across various fields such as medicine, agriculture, and environmental science. Its advent represents not only a notable advancement in genetic manipulation techniques but also plays a vital role in addressing contemporary challenges and ethical dilemmas in biology.
Mechanism of Action
At the heart of CRISPR technology is its mechanism, which utilizes components derived from bacterial immune systems to target and edit specific DNA sequences. The CRISPR system consists of two main parts: the guide RNA and the Cas9 enzyme. The guide RNA is designed to match a specific sequence in the genome, directing the Cas9 enzyme to the precise location where a cut is to be made. Once the cut occurs, cellular repair processes are triggered. These may either lead to disruption of the gene or insert new genetic material if provided.
This system's specificity allows for targeted gene editing, minimizing off-target effects. This precision has vast implications, particularly in areas such as gene therapy, where accurate modifications are essential to avoid unintended consequences in genetic material.
Applications in Research
CRISPR technology has opened new avenues for scientific inquiry and experimentation. In basic research, it allows scientists to create knockout models that help elucidate gene functions by observing phenotypic changes when specific genes are disabled. Furthermore, researchers are utilizing CRISPR to explore gene interactions, pathogenesis of diseases, and potential therapeutic targets.
In medical research, CRISPR's potential to edit genes linked to genetic disorders such as cystic fibrosis or muscular dystrophy is particularly promising. The ability to produce genetically modified organisms enables the study of disease mechanisms in controlled environments, providing critical insights into human physiology and pathology.
Ethical Considerations
With great potential comes considerable ethical responsibility. The use of CRISPR technology raises important questions regarding its application and implications. Concerns primarily revolve around the potential for human germline editing, which could lead to heritable changes in the human genome. This raises issues about consent, accessibility, and the implications of editing traits like intelligence or physical characteristics.
Moreover, the possibility of unintended consequences from gene editing also represents a significant ethical dilemma. An oversight in targeting could result in adverse effects that may not be immediately apparent. Regulatory measures are needed to govern research and clinical applications of CRISPR, ensuring equitable access and responsible use.
"As we advance with CRISPR technology, we must remain vigilant about the ethical frameworks that guide its application. This vigilance is necessary to harness its potential for the betterment of humanity without compromising our moral values."
As CRISPR Technology continues to evolve, it is essential for scientists and policymakers to engage in dialogues that will guide responsible utilization and navigate the complex landscape that this innovation presents.
Gene Sequencing Technologies
Gene sequencing technologies represent a cornerstone in the evolution of genetic understanding. They allow scientists to determine the precise order of nucleotides in an organism's DNA. This capability has profound implications across numerous fields, such as medicine, agriculture, and environmental science. The improvement of sequencing technologies has drastically reduced the cost and time associated with genomic analysis, enabling more researchers and institutions to participate in genetic exploration.
Next-Generation Sequencing
Next-generation sequencing (NGS) is a transformative approach that has revolutionized the field. Unlike traditional methods, NGS can sequence millions of fragments of DNA simultaneously. This high-throughput capacity facilitates extensive genomic studies. As a result, NGS has become integral in projects like the Human Genome Project and various cancer genomics initiatives.
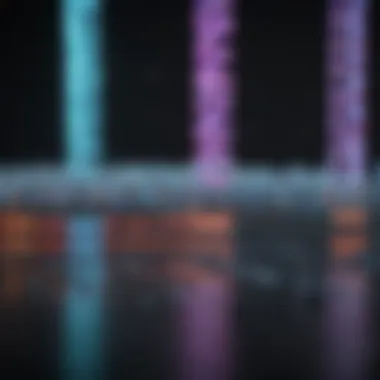
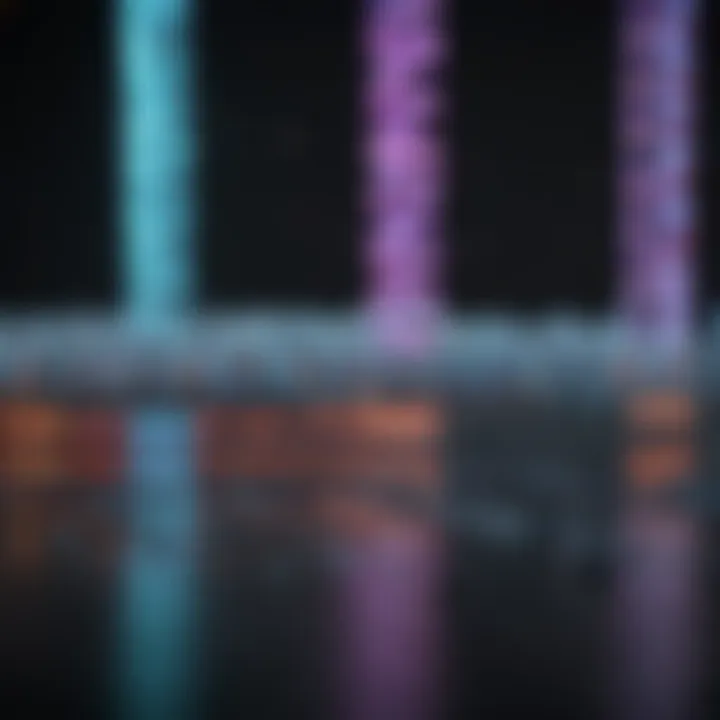
Benefits of Next-Generation Sequencing:
- Cost-Effective: NGS significantly reduces the costs associated with sequencing, making it accessible to broader communities.
- Speed: The capability to sequence large volumes of data quickly allows for timely research outputs.
- Versatility: NGS can sequence various DNA samples, including whole genomes, exomes, and targeted gene panels.
Due to these factors, Next-generation sequencing has become a vital tool in both academic research and clinical applications.
Applications in Genomic Studies
Many applications arise from gene sequencing technologies. NGS, in particular, contributes to various scientific realms. For example, in personalized medicine, physicians can utilize genomic information to tailor treatments to individual patients. This shift towards patient-centered care leads to more effective healthcare outcomes.
Some notable applications include:
- Cancer Genomics: NGS enables the identification of mutations that drive cancer development, informing treatment decisions and developing targeted therapies.
- Infectious Disease Surveillance: Rapid sequencing of pathogens allows health officials to track outbreaks and evolve responses effectively.
- Agricultural Genomics: Sequencing aids in developing disease-resistant crop varieties, enhancing food security.
These examples illustrate how gene sequencing technologies facilitate advancements in understanding health and biology.
Future of Sequencing Technologies
The trajectory of gene sequencing technologies points towards multiple exciting developments. Innovations continue to improve accuracy and accessibility. One promising area under exploration is single-cell sequencing, which may unlock new insights in cellular heterogeneity.
Moreover, the integration of artificial intelligence into sequencing processes enhances data interpretation, facilitating more precise conclusions drawn from genomics.
Potential trends include:
- Real-Time Sequencing: Emerging technologies aim to provide real-time data during sequencing, which could revolutionize clinical diagnostics.
- Long-Read Sequencing: Advances in long-read sequencing will paint a complete picture of complex genomes, leading to better understanding genetic variations.
"The future of gene sequencing holds immense promise, potentially unlocking solutions to some of our most pressing health and environmental challenges."
As these advancements unfold, the potential of sequencing technologies to contribute to innovative research and applications continues to expand.
Molecular Markers in Genetics
Molecular markers play a crucial role in the field of genetics. They provide tangible references that allow researchers to identify specific genes and alleles within a given organism. This ability to pinpoint genetic variations aids in understanding genetic disorders, breeding programs, and conservation efforts. Molecular markers have several benefits, the most significant being their high precision and efficiency in genomic studies. In addition, they assist in the classification and mapping of genomes, paving the way for further exploration and development in genetic research.
Types of Molecular Markers
Molecular markers can be categorized into various types, each serving unique purposes and applications. Common types include:
- Restriction Fragment Length Polymorphisms (RFLPs): These markers are based on the variation in DNA fragment sizes after digestion with restriction enzymes. They have been widely used in genetic mapping.
- Simple Sequence Repeats (SSRs): Also known as microsatellites, SSRs are short, repeating sequences of DNA. They are highly polymorphic, making them useful for genetic diversity studies and parentage analyses.
- Single Nucleotide Polymorphisms (SNPs): SNPs are the most common type of genetic variation among individuals. These markers are crucial for understanding genetic diseases and drug responses in personalized medicine.
- Amplified Fragment Length Polymorphisms (AFLPs): This technique combines the use of restriction enzymes and PCR amplification. AFLPs provide a comprehensive view of genetic variation across multiple regions of the genome.
Each type of marker holds specific advantages, which are useful depending on the research context and goals.
Use in Plant Breeding
Molecular markers have revolutionized plant breeding practices by enabling the selection of desirable traits with greater accuracy. Farmers and breeders can bypass traditional methods that rely on phenotypic observation. For instance, using SNP markers, breeders can make informed decisions about which plants to cross for genetic improvements such as yield and disease resistance.
Advantages of using molecular markers in plant breeding include:
- Faster Selection: Molecular techniques allow for faster identification of desirable traits compared to conventional breeding methods.
- Increased Precision: Breeders can target specific genes related to traits of interest, leading to improved outcomes.
- Enhanced Genetic Diversity: Molecular markers facilitate the preservation and utilization of genetic resources.
Applications in Conservation Biology
Molecular markers are also essential in conservation biology. They help in assessing genetic diversity within endangered species, which is vital for effective conservation strategies. By analyzing genetic variation, researchers can identify populations at risk of inbreeding and decline.
Applications in conservation include:
- Monitoring Genetic Health: Tracking the genetic variability within populations helps in assessing their ability to adapt to environmental changes.
- Identifying Ecological Corridors: Molecular data aid in planning the establishment of corridors for gene flow between isolated populations.
- Understanding Evolutionary Relationships: Researchers can use molecular markers to reconstruct the evolutionary history of species, informing conservation priorities.
Impact on Medicine
The impact of genetic tools on medicine is profound and multifaceted. Genetic research has significantly altered how we understand diseases, their mechanisms, and their treatments. This section focuses on how genetic tools enhance medical practice through genetic testing, gene therapy innovations, and personalized medicine. These advancements not only improve patient outcomes but also reshape public health strategies and ethical considerations in medicine.
Genetic Testing
Genetic testing involves analyzing an individual's DNA to identify genetic predispositions to certain diseases. This practice improves disease prevention and management through early detection and risk assessment. By identifying gene mutations, physicians can recommend tailored screening programs and preventive measures.
Some key advantages of genetic testing include:
- Early Diagnosis: Enables timely interventions before symptoms appear.
- Risk Assessment: Helps patients understand their likelihood of developing specific conditions, assisting in making informed lifestyle or medical choices.
- Informed Family Planning: Provides prospective parents with information that can affect reproductive decisions.
However, ethical concerns arise with genetic testing, such as privacy issues and the psychological impact of test results. Addressing these considerations is crucial for the responsible implementation of testing.
Gene Therapy Innovations
Gene therapy has emerged as a revolutionary approach in treating genetic disorders. This technique involves modifying or replacing faulty genes with the aim of curing or alleviating disease. Recent innovations in gene delivery systems, such as viral vectors, have enhanced the efficiency of these treatments.
Several areas where gene therapy is transforming medical practice include:
- Treatment of Rare Diseases: Allows targeting of specific genetic conditions that have limited treatment options.
- Potential Cure for Genetic Disorders: Offers hope for conditions like cystic fibrosis and sickle cell anemia.
- Improvement of Standard Treatments: Enhances existing therapies by addressing the underlying genetic causes.
Despite the promise, there are challenges, including the high costs of therapies and potential long-term effects that are not yet well understood.
Personalized Medicine
Personalized medicine represents the shift from one-size-fits-all treatment approaches to more individualized strategies, tailored to the genetic profile of each patient. This method relies heavily on genetic information to guide treatment decisions, improving the effectiveness and reducing the risk of adverse effects.
Key elements of personalized medicine include:
- Tailored Drug Formulation: Understanding how genetic variations affect drug metabolism allows healthcare providers to customize medication doses.
- Targeted Therapies: Focus on specific molecular targets, increasing treatment precision.
- Monitoring Treatment Response: Allows ongoing adjustments based on individual responses.
The integration of genetic tools in personalized medicine is transforming the way healthcare providers deliver treatment, offering more effective solutions to patients. As we advance, a thorough understanding of ethical, social, and legal challenges remains paramount in the progression of genetic medicine.
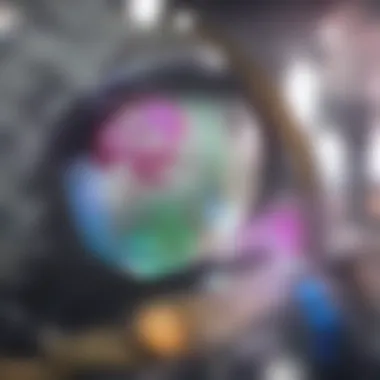
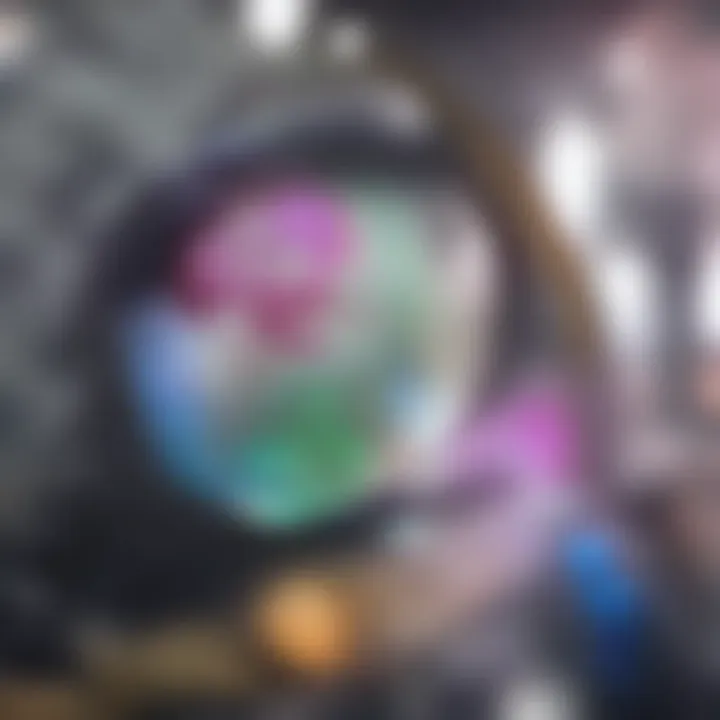
"The future of medicine lies in understanding it as a genetic science." - Emergent Thought
In summary, genetic tools are reshaping medicine fundamentally. They provide enhanced methods for diagnosis, treatment, and prevention of illness. Through thoughtful integration of ethical considerations, the field continues to expand, offering promising directions for research and practice.
Applications in Agriculture
The integration of genetic tools in agriculture marks a pivotal evolution in the way crops are developed and managed. This sector, crucial for global food security, faces immense challenges including climate change, population growth, and the decreasing availability of arable land. Through innovative genetic techniques, agriculture can enhance productivity while addressing these pressing issues.
Genetic Modification Techniques
Genetic modification techniques, such as transgenic strategies, allow scientists to introduce specific genes into crops to impart desired traits. This may include increased resistance to pests, enhanced nutritional content, or improved tolerance to harsh environmental conditions. Notable examples include Bt cotton and Bt maize, which incorporate a gene from the bacterium Bacillus thuringiensis, providing inherent resistance to certain herbivorous pests.
Techniques like CRISPR/Cas9 also enable precise modifications which can enhance traditional breeding practices. This precision reduces the time and resources needed to develop new crop varieties, making it possible to respond more swiftly to evolving agricultural challenges. Understanding the molecular pathways through which these modifications occur is essential for the advancement of these technologies in agricultural practices.
Crop Improvement Strategies
Crop improvement strategies utilize genetic tools to enhance the resilience and performance of crops. Marker-assisted selection is one such strategy, where molecular markers linked to desirable traits guide the breeding process. This method streamlines the identification of superior genotypes and promotes faster breeding cycles.
Additionally, genomic selection techniques enable breeders to predict the performance of untested lines based on their genetic information. By combining various methods, such as genomic selection and traditional approaches, agricultural scientists can develop crops that not only yield more but also require fewer inputs.
"Genetic modifications in crops have the potential to significantly alleviate world hunger by ensuring food security through increased yields and resilience."
Sustainability and Environmental Impact
The use of genetic tools in agriculture also raises important discussions around sustainability and environmental impact. Genetically modified crops can reduce the dependence on chemical pesticides, leading to lower ecological footprints. Furthermore, crops engineered for drought resilience can be cultivated in less favorable climates, minimizing water use.
Despite these benefits, there are concerns regarding the long-term effects of genetically modified organisms (GMOs) on biodiversity and ecosystem health. It is important to implement comprehensive assessments to evaluate these risks. Research is ongoing to explore sustainable practices that combine genetic advancements with environmental stewardship, fostering a balance that benefits both farmers and ecosystems.
Role in Environmental Science
The role of genetic tools in environmental science is foundational and multifaceted. As environmental issues intensify, genetic technologies can provide innovative solutions to significant challenges. Environmental scientists leverage these tools to understand ecosystems, address pollution, and conserve biodiversity. The integration of genetic technologies in environmental science allows for a more precise and informed approach toward managing natural resources and restoring ecosystems. Whether through bioremediation or biodiversity conservation, these advancements are commendable and urgently needed.
Bioremediation Techniques
Bioremediation is a vital application of genetic tools in environmental management. It involves utilizing living organisms, often microorganisms, to degrade environmental pollutants like oil spills or heavy metals. Genetic engineering has enabled scientists to enhance the capabilities of these organisms. For instance, modified bacteria can be engineered to break down toxic substances like polychlorinated biphenyls (PCBs) or to metabolize hydrocarbons present in oil spills.
Many existing bioremediation techniques are effective but can be limited by the natural potential of the organisms involved. Genetic modifications can expand their environmental range, improve survival rates in contaminated sites, and increase the rate at which pollutants are broken down. This efficiency makes bioremediation a more attractive and effective method than traditional clean-up strategies, which are often costly and time-consuming.
"Genetic modifications in bioremediation can significantly reduce the time required for cleanup, making it a proactive approach in tackling pollution."
Additionally, bioremediation can lead to less waste, promoting sustainability. By focusing on these methods, scientists can restore contaminated sites more effectively, aiding in overall environmental health.
Biodiversity Conservation Efforts
Biodiversity conservation is another critical area where genetic tools have substantial impact. As habitats disappear due to human activities and climate change, conserving genetic diversity within species becomes essential. Genetic tools offer opportunities to assess and manage the genetic health of endangered populations. Tools such as DNA barcoding and genetic markers allow scientists to monitor genetic diversity and understand population structures.
Understanding the genetic variation within a species helps in developing strategies to enhance resilience against changes in the environment. For example, genetic diversity can provide insights into a populationsβ adaptability to climate fluctuations or disease resistance. The use of genetic tools aids conservationists in making informed decisions about breeding programs or habitat restoration projects aimed at protecting endangered species.
Moreover, genetic techniques contribute to efforts in rewilding and habitat restoration. By understanding the genetic makeup of plants and animals, restoration projects can select appropriate native species for reintroduction or reforesting efforts, ensuring that the restored ecosystems are genetically diverse and capable of sustaining themselves long-term.
Bioinformatics and Data Analysis
Bioinformatics and data analysis are pivotal in understanding and interpreting complex biological data. These fields have gained substantial significance with the rise of genetic tools, particularly in genomic research. By processing large datasets, researchers can uncover insights that were once difficult to access. Here, we explore three main facets of bioinformatics: computational genomics, data mining techniques, and the role of machine learning in genetics.
Computational Genomics
Computational genomics involves the application of computational methods to analyze genomic data. The increasing volume of genetic information being generated by techniques like next-generation sequencing necessitates advanced software tools. These tools enable scientists to map genomes quickly and accurately. They also facilitate the identification of genetic variations responsible for various traits and diseases.
Some essential applications of computational genomics include:
- Genome assembly: Bringing together short DNA sequences into a single, coherent sequence.
- Variant calling: Identifying differences between sequences to find potential mutations.
- Functional annotation: Determining the function of genes based on their sequence data.
The ability to perform these tasks effectively is vital for progressing in fields like personalized medicine and evolutionary biology. The precision achieved through computational methods leads to improved accuracy in genetic research.
Data Mining Techniques
Data mining in genetics involves extracting useful information from large datasets. These techniques can uncover patterns and relationships that are not apparent through traditional analysis methods. With the boom in high-throughput sequencing technologies, data mining becomes indispensable.
Key data mining techniques used in genetic research include:
- Clustering: Grouping similar data points for better understanding of genetic structures.
- Classification: Assigning labels to genetic data based on predefined criteria.
- Regression analysis: Determining relationships between genetic variations and phenotypic expressions.
Data mining allows researchers to interpret genetic data in innovative ways, improving our understanding of gene function and interaction.
The implications of data mining extend beyond research. They are also crucial in drug discovery and disease prediction, leading to more targeted therapies.
Machine Learning in Genetics
Machine learning increasingly plays a role in genetics as it offers powerful methods for data interpretation and analysis. Algorithms can learn from genetic data and improve their predictions over time. This capability enables researchers to handle complexities found in biological data.
Applications of machine learning in genetics include:
- Predictive modeling: Anticipating genetic predispositions to diseases based on various factors.
- Genomic feature selection: Identifying significant genetic markers that contribute to diseases or traits.
- Image analysis: Analyzing images from histology or microscopy to correlate genetic data with visual phenotypes.
Machine learning algorithms enhance the speed and efficiency of research, paving the way for breakthroughs in genomics and biotechnology.
In summary, bioinformatics and data analysis form the backbone of modern genetic research. Understanding computational genomics, data mining techniques, and the use of machine learning tools is essential for anyone looking to advance their knowledge or career in this exciting and rapidly evolving field.
Challenges in Genetic Research
The landscape of genetic research is evolving rapidly, propelled by various technological advances. However, this field is not without challenges. Understanding these challenges is critical for researchers, policy makers, and the wider public. Regulatory issues, socioeconomic barriers, and ethical considerations play vital roles in shaping the progress and acceptance of genetic technologies.
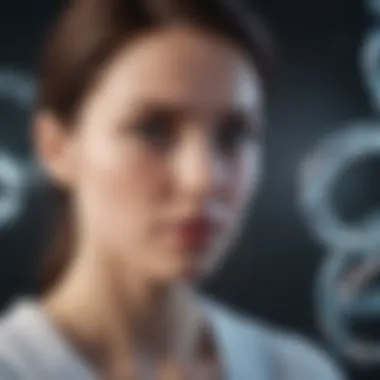
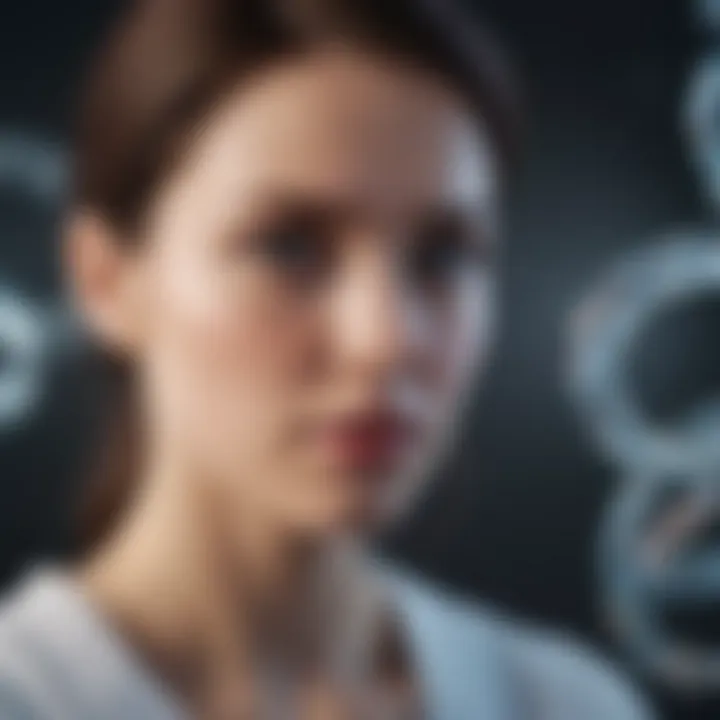
Regulatory Issues
Regulatory frameworks governing genetic research vary significantly across regions. In many cases, these regulations are outdated, creating hurdles for researchers who are trying to navigate through complex approval processes. For example, the use of CRISPR technology often involves lengthy consultations with regulatory bodies. This can delay essential research that could lead to breakthroughs in treatments or agricultural enhancements.
Moreover, different countries have distinct regulations regarding gene editing in humans, plants, and animals. This disparity can limit collaborative efforts and slow down the pace of innovation. Additionally, enforcement of regulations can sometimes be inconsistent, raising questions about compliance and the ethical standards of research conducted in various environments.
"A clear and consistent regulatory framework is essential for fostering innovation while ensuring safety and ethics."
As technologies like gene editing continue to develop, it is imperative for regulatory bodies to keep pace. They must strike a balance between encouraging scientific advancement and protecting public health and safety.
Socioeconomic Barriers
Socioeconomic factors significantly affect access to genetic research and its benefits. Disparities in income and education can create gaps in access to advanced genetic technologies. Individuals in lower socioeconomic brackets may not have the same access to genetic testing or therapies as those in higher income brackets. This raises ethical concerns about fairness and justice in healthcare.
Furthermore, there is often a lack of awareness or understanding of genetic tools among underprivileged communities. Educational resources about genetic technologies may not be readily available, leading to a cycle of ignorance that compounds the problems of access and equity.
Investment in genetic research is often concentrated in wealthier nations, limiting opportunities for scientists in developing countries. This can result in a brain drain, where talented researchers leave their home countries for better opportunities abroad.
Ethics and Public Perception
The ethical landscape surrounding genetic research is complex. There are numerous concerns about the implications of gene editing, especially when it comes to making changes to the human genome. These concerns include the potential for unintended consequences and the moral questions surrounding the "playing God" argument. Public perception plays a key role in determining how such technologies are adopted or rejected.
These ethical concerns often result in resistance from the public and advocacy groups. Misinformation and fear can overshadow the potential benefits of genetic research, leading to stringent regulations and policies that stifle innovation. Engaging the public in informed discussions about genetic technologies is crucial for building trust and understanding. Researchers and policymakers must work together to communicate the science effectively, debunk myths, and highlight the positive impacts of genetic tools on society.
In summary, the challenges in genetic research are multifaceted and require a thoughtful approach. Regulatory issues, socioeconomic barriers, and ethical considerations all play important roles. Addressing these challenges is critical for ensuring that genetic advancements can be fully realized and equitably distributed in society.
Future Directions in Genetic Tools
The future of genetic tools is of critical importance in this article. As the landscape of genetic research and technology continues to evolve, understanding the trajectory of these advancements is essential. Future directions in genetic tools encompass the interplay of innovation, emerging trends, and collaborative efforts across disciplines. Analyzing these elements brings to light the potential benefits and considerations that researchers, educators, and professionals must navigate moving forward.
Innovation and Emerging Trends
Innovations in genetic tools are paving pathways previously deemed unreachable. The development of more precise and efficient methods of gene editing, such as CRISPR-Cas9 modifications, offers groundbreaking potential in various fields, including therapeutic applications and agriculture. Not only does this technology aim to enhance efficiency, but it also focuses on improving the safety and efficacy of genetic interventions.
Some key areas of innovation include:
- Synthetic Biology: This area combines biology and engineering, giving rise to novel organisms or biological systems that perform specific functions. Advances here can lead to the development of new therapies and sustainable biological solutions.
- Gene Drives: Gene drives facilitate the dissemination of certain genetic traits across populations, which can be utilized for pest control or to modify disease vectors like mosquitoes. This innovative tool opens discussions on ecological impacts and regulatory frameworks.
- Single-cell Genomics: This emerging trend allows researchers to study individual cells, yielding insights into cellular diversity and behavior. Such technology is crucial in understanding complex diseases.
The continuous evolution of these tools is crucial for addressing challenges in genetics, whether related to human health, agriculture, or environmental conservation.
Cross-disciplinary Collaboration
The complexity of genetic research calls for a collaborative approach that spans multiple disciplines. Biologists, geneticists, computer scientists, ethicists, and policy makers must work together to ensure the responsible and efficient use of genetic tools. Cross-disciplinary collaboration can accelerate discoveries and innovations by bringing together different expertise and perspectives.
Key considerations in fostering collaboration include:
- Data Sharing: Open access to genetic data can promote advances in research and innovation. Collaborations that focus on sharing findings may lead to breakthroughs that individual entities could not achieve alone.
- Educational Partnerships: Institutions can benefit from partnerships that integrate genetics education into broader curriculums. This prepares the next generation of researchers and professionals to tackle complex genetic problems.
- Ethics and Policy Formation: Working together can ensure that ethical standards evolve alongside technological advancements. Discussion among disciplines can establish responsible governance regarding the use of genetic technologies.
"Collaborative efforts in genetics can lead to greater understanding and unprecedented breakthroughs."
In summary, the future of genetic tools is rich with opportunities. By embracing innovation and nurturing cross-disciplinary collaboration, researchers can enhance the impact of genetic technologies. Both elements are essential for navigating the complex ethical landscape while meeting societal needs.
Culmination
The importance of the conclusion section in this article lies in its role as a synthesis of the advancements in genetic tools and their multifaceted impact on various fields. This article has explored a wide range of genetic technologies, such as CRISPR, gene sequencing, and molecular markers. Each of these tools plays a crucial role in shaping contemporary research methodologies, particularly in areas like medicine, agriculture, and environmental science.
A key consideration in this discussion is the ethical implications that arise from these technologies. As genetic tools become more advanced, the questions surrounding their use and regulation become more pronounced. The potential for misuse or unintended consequences raises significant ethical debates, necessitating ongoing conversations among scientists, ethicists, and the public. To encapsulate a few important points:
- Technological Evolution: The evolution of genetic research tools is not just about biological advancements; it also involves a shift in how scientists approach problems and innovate solutions.
- Accessibility and Regulation: The future of genetic research depends on balancing innovation with proper regulation to ensure these technologies serve societyβs best interests.
- Interdisciplinary Approaches: Collaboration across various scientific fields can lead to more comprehensive strategies in utilizing genetic tools effectively and ethically.
In summary, this article highlights crucial developments in genetic tools, emphasizing their transformative potential while recognizing the ethical responsibilities they impose.
Summary of Key Insights
Throughout this article, several key insights have emerged, contributing to a deeper understanding of genetic tools:
- CRISPR as a Game Changer: CRISPR technology stands out for its precision and efficiency in gene editing. Its applications range from basic research to advanced therapeutic techniques.
- Next-Generation Sequencing: This technology has vastly improved our ability to analyze genetic information, paving the way for personalized medicine and detailed genomic studies.
- Impact on Agriculture: Genetic modification techniques are crucial for achieving sustainability in agriculture, enhancing crop resilience against climate change.
- Bioinformatics: The integration of bioinformatics tools is essential for managing the massive data generated in genetic research, enabling efficient data analysis and interpretation.
Key Points to Remember
- The rapid pace of advancements in genetic research tools continues to reshape our understanding of genetics.
- Ethical considerations and public perception significantly influence the trajectory of genetic technologies.
- Cross-disciplinary collaboration enhances the potential benefits of genetic tools while mitigating risks.
The Path Forward
Looking ahead, the future of genetic tools is filled with possibilities. Advancements in this field are expected to accelerate, influenced by several factors:
- Innovation: Ongoing research and development will lead to the emergence of new techniques and technologies. For instance, advancements in gene editing could enable more complex interventions in genetic diseases.
- Ethical Frameworks: Developing robust ethical frameworks will be essential as genetic tools evolve. It is crucial to establish guidelines that balance innovation with societal concerns.
- Global Collaboration: The future landscape of genetic research will benefit from increased collaboration across borders, reducing knowledge silos and fostering shared goals in research applications.
"The future of genetic tools is not just about enhancing capabilities; it is also about addressing the profound ethical and societal implications that come with them."
Citing Source Material
Citing source material is imperative to uphold the integrity of scientific work. It ensures that original ideas and findings are acknowledged, preventing plagiarism and promoting the work of others in the field. When researchers cite studies, articles, and data, they offer readers a pathway to validate the information presented.
Additionally, the reference list acts as a tool for deeper learning. It reflects the landscape of the existing knowledge, guiding future research endeavors. Importantly, various citation styles exist, such as APA, MLA, and Chicago, each tailored for different contexts. Choosing the right format is essential, as each has its own rules.
Recommended Reading
For those looking to delve deeper into genetic tools and their applications, several resources provide valuable insights:
- Molecular Biology of the Cell by Alberts et al.
This textbook offers a comprehensive overview of cellular and molecular biology, with sections devoted to genetic research and technology. - The Double Helix: A Personal Account of the Discovery of the Structure of DNA by James Watson
A fascinating narrative that explores the history of DNA research, shedding light on the scientists behind the discoveries. - Genomes 4 by T.A. Brown
This book provides an up-to-date discussion of sequencing technologies and their implications in genetics.
Through these texts, readers can enhance their understanding of not just genetic tools but also the broader context of how these advances continue to shape research and technology.
"Research is what Iβm doing when I donβt know what Iβm doing."
β Wernher von Braun
By engaging with reputable sources, researchers and students can ensure their work is grounded in established knowledge. This dedication to research fidelity ultimately strengthens the integrity of genetic science.