Understanding the Impact of RNA Biology Today
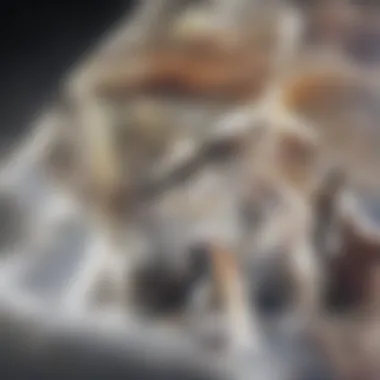
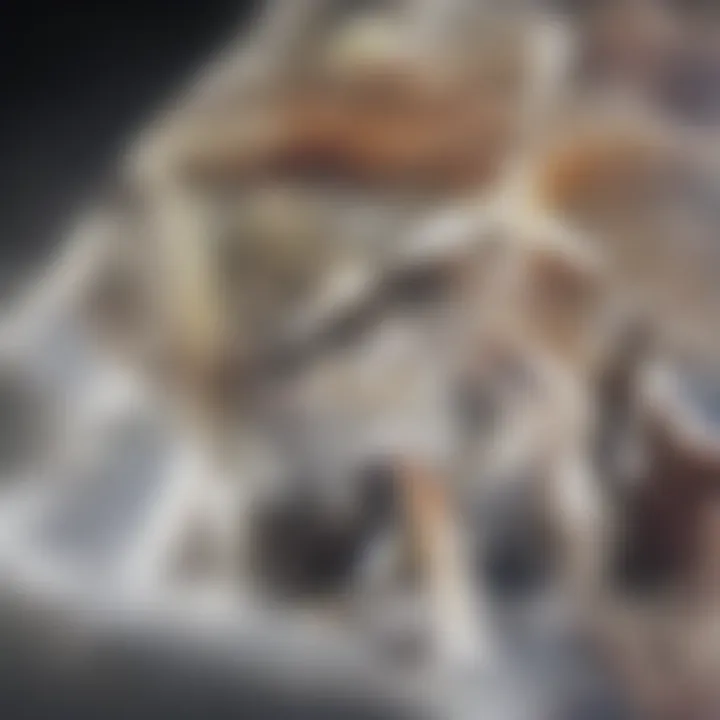
Intro
RNA biology plays an essential role in understanding the complexity of life and disease. The significance of RNA extends far beyond the traditional view of acting merely as a messenger. Current research emphasizes multiple functions that RNA performs within the cellular environment.
Researchers are now uncovering the diverse roles of various types of RNA, including messenger RNA (mRNA), ribosomal RNA (rRNA), transfer RNA (tRNA), and regulatory RNAs such as microRNA. These elements are central in processes such as gene expression, cellular signaling, and even protein synthesis. This shift in focus towards RNA opens new pathways for understanding biological mechanisms that underpin health and disease.
Recent advancements in technologies like RNA sequencing and CRISPR-Cas9 gene editing have propelled our understanding forward. Such tools are instrumental in unraveling the complexities of RNA and its interactions within biological networks. As researchers delve deeper, the insights gained are proving invaluable in fields such as oncology, neurology, and infectious diseases.
Understanding RNA biology is increasingly relevant in the context of personalized medicine and therapeutic development. By exploring how RNA functions can lead to targeted interventions, we can hope for better management of diseases that have proven challenging thus far.
In the sections that follow, the article will explore these themes in depth. Key findings from recent studies will be presented, alongside a discussion of their implications for the future of RNA research.
Prelude to RNA Biology
RNA biology has become an essential area of study in contemporary research, particularly because of its multifaceted roles within cellular processes. Unlike DNA, which serves as a static repository of genetic information, RNA is dynamic and participates directly in various biochemical activities. Understanding RNA is crucial not only for comprehending fundamental biological mechanisms but also for developing innovative therapeutic strategies against diseases.
The study of RNA encompasses its diverse types, functions, and the regulatory networks it is involved in. This knowledge is vital for students, researchers, educators, and professionals aiming to grasp the complexities of cellular machinery. By exploring RNA biology, one can uncover insights into genetic regulation, protein synthesis, and even the implications of RNA in disease pathology.
As researchers continue to unveil the intricate functions of RNA, its significance expands, particularly in the context of health and disease. This introduction sets the stage for a deeper understanding of RNA, paving the way for discussions on its historical significance and basic concepts.
Historical Context
The exploration of RNA biology began in the mid-20th century, following the discovery of the structure of DNA by James Watson and Francis Crick in 1953. Initially, RNA was thought to be merely a messenger that transferred genetic information from DNA to proteins. However, the subsequent discoveries revealed that RNA plays critical roles in various cellular activities, including gene regulation, enzyme activity, and cellular signaling pathways.
One landmark event was the discovery of ribozymes in the 1980sβRNA molecules that can catalyze chemical reactions. This finding shifted the view of RNA from a simple messenger to an active participant in biological processes. Further research in the 1990s into non-coding RNAs, such as microRNAs and long non-coding RNAs, highlighted the diverse functionalities of RNA beyond protein coding.
This historical perspective underscores how RNA biology has evolved from a simplistic understanding to a complex field with emerging relevance across multiple scientific disciplines.
Basic Concepts of RNA
To appreciate the significance of RNA, it is important to understand its basic structure and different types. RNA is composed of nucleotides, each made up of a ribose sugar, a phosphate group, and a nitrogenous base. The four bases found in RNA are adenine, uracil, cytosine, and guanine.
The major types of RNA include:
- Messenger RNA (mRNA): Carries genetic information from DNA to the ribosome, where it serves as a template for protein synthesis.
- Ribosomal RNA (rRNA): Forms the structural and functional core of ribosomes, the cellular machinery responsible for assembling proteins.
- Transfer RNA (tRNA): Brings amino acids to the ribosome during protein synthesis, matching them with the corresponding codon on the mRNA strand.
- Non-coding RNA: Includes various RNA types that do not code for proteins but have regulatory roles, such as microRNA and long non-coding RNA.
Understanding these basic concepts is essential for grasping the more complex interactions and functions of RNA within biological systems. As research in this area progresses, the implications of RNA biology continue to surface, heralding new avenues for exploration and discovery.
Types of RNA
Understanding the different types of RNA is crucial for grasping the vast landscape of RNA biology. Each RNA type plays a distinct role in cellular functions, influencing various biological processes. These roles significantly affect research in genetics, molecular biology, and biotechnology. As research progresses, we see that RNA types are not just fulfilling their basic functions but are also involved in complex regulatory mechanisms that have broader implications for health and disease. This section highlights the importance of four main RNA types: messenger RNA (mRNA), ribosomal RNA (rRNA), transfer RNA (tRNA), and non-coding RNA.
Messenger RNA (mRNA)
Messenger RNA (mRNA) is vital for encoding genetic information that is translated into proteins. It serves as the intermediary between DNA and protein synthesis. The significance of mRNA lies in its role in conveying genetic instructions from the nucleus to the ribosomes, where proteins are produced. This transfer is essential for cellular function and regulation.
In contemporary research, the study of mRNA has gained prominence, especially with the advent of mRNA vaccines like those from Pfizer and Moderna, which have played a critical role in the response to the COVID-19 pandemic. The use of synthetic mRNA in therapeutic applications shows promise for treating a wide array of diseases.
Ribosomal RNA (rRNA)
Ribosomal RNA (rRNA) is a fundamental component of ribosomes, the cellular machinery responsible for protein synthesis. It comprises the structural and functional core of these complexes, facilitating the translation process from mRNA to protein.
Research has highlighted the importance of rRNA in understanding the intricacies of protein synthesis. Mutations or alterations in rRNA can lead to various diseases. The study of rRNA thus offers insights into cellular function, evolution, and dysfunction in disease processes.
Transfer RNA (tRNA)
Transfer RNA (tRNA) is instrumental in the translation of mRNA into proteins by transporting specific amino acids to the growing polypeptide chain. Each tRNA molecule is linked to a specific amino acid and recognizes corresponding codons on the mRNA. The accuracy of tRNA in matching amino acids with codons is critical for the fidelity of protein synthesis.
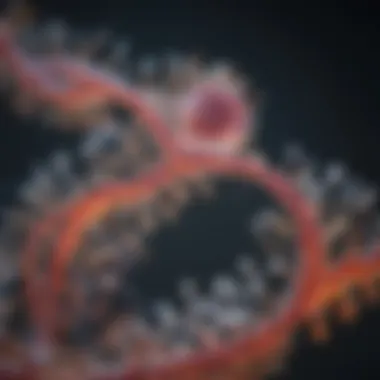
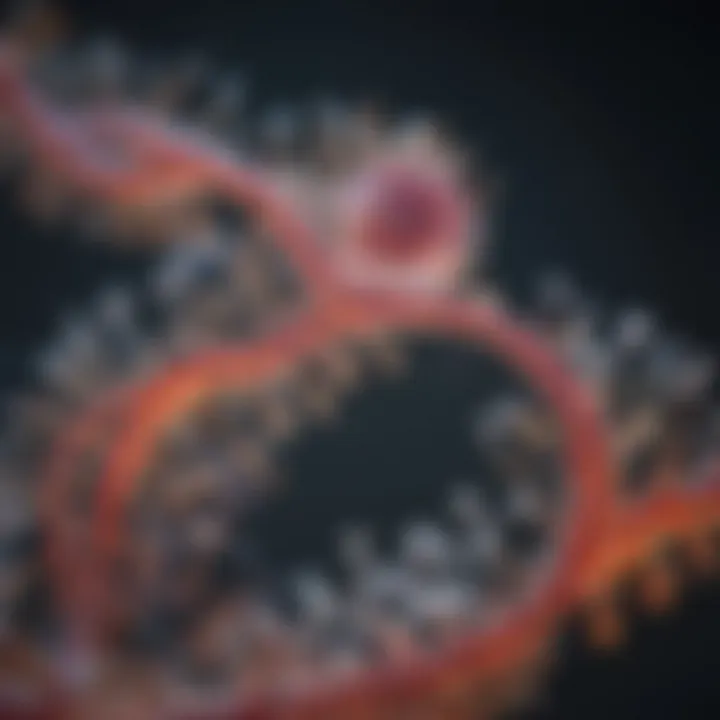
In scientific research, tRNA's role extends beyond translation. It is involved in regulatory mechanisms through its interaction with various molecules. As such, understanding tRNA's functions provides a deeper insight into cellular regulation and potential pathways for therapeutic interventions.
Non-Coding RNA
Non-coding RNA (ncRNA) is an umbrella term for RNA molecules that do not encode proteins. This category includes several subclasses that play significant roles in regulating gene expression and maintaining cellular homeostasis. Within non-coding RNA, two critical classes are microRNA (miRNA) and long non-coding RNA (lncRNA).
MicroRNA (miRNA)
MicroRNA (miRNA) contributes to the regulation of gene expression and is a well-studied class of non-coding RNA. These small molecules control the expression levels of target mRNAs, often leading to degradation or repression of translation. This regulatory function is crucial in controlling various biological processes, including development, differentiation, and apoptosis.
One key characteristic of miRNAs is their ability to target multiple mRNAs, making them influential in complex regulatory networks. They are a beneficial choice for researchers; their role in cancer and other diseases highlights their potential as therapeutic targets.
The unique feature of miRNA is their conservation across species, suggesting evolutionary importance. However, their regulation can lead to challenges in therapy, as the same miRNA may influence multiple pathways.
Long Non-Coding RNA (lncRNA)
Long non-coding RNA (lncRNA) refers to a diverse group of RNA molecules longer than 200 nucleotides that possess regulatory capabilities. LncRNAs are involved in various processes, including chromatin remodeling, transcriptional regulation, and post-transcriptional control.
Their key characteristic lies in their ability to interact with DNA, RNA, and proteins, serving as scaffolds or decoys. This interaction influences gene expression and chromatin structure, which are critical in maintaining cellular homeostasis. LncRNAs are increasingly recognized for their notable roles in disease contexts, especially in cancer.
One unique aspect of lncRNA is their tissue-specific expression patterns, indicating their tailored regulatory roles in different contexts. While they present substantial research opportunities, challenges arise from their complexity and the need for advanced methodologies to study their functions effectively.
Understanding the diverse roles of RNA types opens avenues for innovative research and therapeutic strategies, emphasizing the need to investigate their functions comprehensively.
In summary, the various types of RNA carry unique functions that are fundamental to biological processes and contemporary research. Each type, from mRNA to non-coding RNA, plays an invaluable role in our understanding of cellular mechanisms and the development of therapeutic interventions.
RNA Synthesis and Processing
RNA synthesis and processing are central to understanding how genetic information is utilized within a cell. The process describes how RNA molecules are created from DNA templates and subsequently modified before they perform their roles in various biological functions. This topic is crucial because it underscores the intricate mechanisms that govern gene expression and regulation. Furthermore, the efficiency and accuracy of RNA synthesis and processing greatly influence cellular functions and overall organismal development.
Transcription Mechanisms
Transcription is the first step in the process of RNA synthesis. It involves the generation of RNA from a DNA template, wherein specific genes are transcribed into messenger RNA (mRNA). The core enzyme responsible for this process is RNA polymerase. This enzyme binds to a region of the DNA known as the promoter, initiating the transcription. As RNA polymerase moves along the DNA strand, it unwinds the double helix, synthesizing RNA by adding nucleotides complementary to the DNA template. The accuracy of this process is significant, as any errors can lead to the production of malfunctioning proteins.
The transcription can be regulated through various mechanisms, such as the presence of transcription factors. These proteins can enhance or repress the transcription of specific genes, allowing for precise control over gene expression. Understanding these regulatory networks is important, as they play a vital role in development and responses to environmental changes.
Post-Transcriptional Modifications
Once RNA is synthesized, it often undergoes several post-transcriptional modifications before it can perform its functions. These modifications include capping, polyadenylation, and splicing. Each of these steps is essential for ensuring the stability and translatability of the mRNA.
- Capping: The addition of a 7-methylguanylate cap at the 5' end of the mRNA. This cap protects the mRNA from degradation and is crucial for ribosome binding during translation, enhancing the efficiency of protein synthesis.
- Polyadenylation: The addition of a poly(A) tail at the 3' end. This tail also protects the mRNA and aids in the export of the mRNA from the nucleus to the cytoplasm, where translation occurs.
- Splicing: The process of removing non-coding regions (introns) from the pre-mRNA transcript. This refinement allows for the production of mature mRNA that only contains coding sequences (exons) that will be translated into proteins.
These modifications are critical for the functional capacity of RNA molecules in the cell. Any irregularities in these steps can lead to diseases, including cancer and genetic disorders. Thus, a thorough understanding of RNA synthesis and processing is paramount in contemporary molecular biology and therapeutic research.
Functions of RNA
RNA plays a crucial role in a variety of cellular processes that define biological function. Understanding the functions of RNA helps highlight its significance in contemporary research, particularly in areas such as genetics, cellular biology, and molecular medicine. The properties of RNA allow it not just to transmit genetic information but also to actively participate in the regulation and maintenance of cellular functions. Here we will discuss three primary functions of RNA: protein synthesis, gene regulation, and its catalytic abilities.
Protein Synthesis
Protein synthesis is one of the most vital functions of RNA. It is the process through which cells build proteins based on the genetic code stored in DNA. Messenger RNA (mRNA) carries genetic information from the DNA in the nucleus to the ribosomes in the cytoplasm, where proteins are synthesized. This translation process involves various types of RNA, including
- Ribosomal RNA (rRNA): This type of RNA is a fundamental component of ribosomes, the cellular machinery responsible for protein synthesis. rRNA facilitates the binding of tRNA and mRNA during translation.
- Transfer RNA (tRNA): tRNA helps bring the correct amino acids to the ribosome in accordance with the mRNA sequence. Each tRNA molecule is specific to one amino acid and recognizes the corresponding codon on the mRNA.
In summary, without RNA, the process of protein synthesis would not occur, leading to disrupted cellular functions.
Gene Regulation
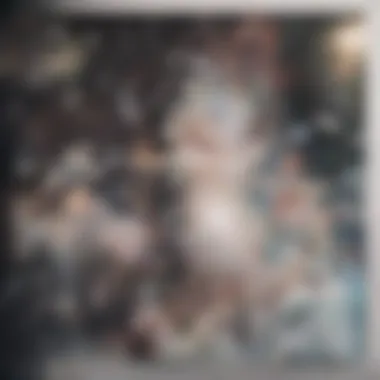
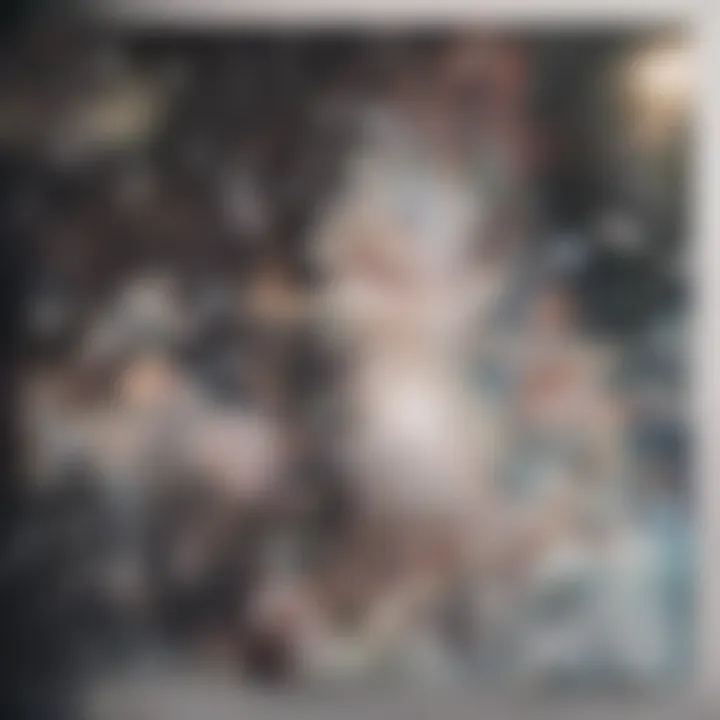
The role of RNA in gene regulation is increasingly understood as researchers delve deeper into the complexities of genetic expression. Non-coding RNAs, particularly microRNAs (miRNAs) and Long Non-Coding RNAs (lncRNAs), are instrumental in modulating gene expression. For example, miRNAs can bind to target mRNAs, leading to their degradation or inhibition of translation. This regulatory mechanism plays a key part in development, differentiation, and response to cellular signals.
Research shows that disruptions in these regulatory mechanisms can lead to diseases, including cancer. Therefore, understanding gene regulation through RNA is essential for developing targeted therapies.
RNA as Catalysts
In addition to its roles in protein synthesis and gene regulation, RNA can also act as a catalyst. This property challenges the long-held view that only proteins can function as enzymes. Ribonuclease P is one example of an RNA molecule that catalyzes the cleavage of precursor molecules to form mature tRNA. Another example is the ribozymes, which are RNA molecules capable of catalyzing biochemical reactions without protein assistance.
This catalytic capability of RNA has significant implications for biological systems. It suggests that RNA might have played a more critical role in the early stages of life's evolution than previously thought. Furthermore, understanding ripozymes can open new pathways in biotechnology and therapeutic interventions.
"The multifaceted roles of RNA extend well beyond information transmission, rendering it a fundamental component in the study of biology and medicine."
Overall, the functions of RNA are integral to understanding how cells operate, regulate gene expression, and evolve. With ongoing advancements in RNA research, it is clear that RNA biology will continue to offer insights that are pivotal to contemporary scientific inquiry.
RNA in Health and Disease
RNA plays a crucial role in various biological processes that affect health and disease. Understanding RNA's contributions can reveal new insights into diagnostics and therapeutics. For instance, its involvement in gene expression regulation can shift our perspective on how diseases develop and manifest.
Role in Cancer
Cancer cells often exhibit dysregulated RNA expression patterns. Abnormal levels of messenger RNA (mRNA) can lead to the production of oncogenic proteins that drive tumorigenesis. Tools such as RNA sequencing enable researchers to identify specific RNA signatures for different cancer types.
The role of non-coding RNAs, including microRNAs and long non-coding RNAs, cannot be ignored. These RNA types can function as oncogenes or tumor suppressors, influencing the cancer progression. For example, certain microRNAs can downregulate genes responsible for suppressing tumors, while others may promote survival and proliferation of cancer cells.
Clinical applications are emerging, most notably in targeted therapies. Understanding how RNA interacts with cancer pathways can lead to novel treatment approaches, making RNA a focal point in cancer research.
Involvement in Genetic Disorders
Genetic disorders often arise from mutations affecting the RNA level. For instance, mutations in genes coding for proteins can produce aberrant mRNA, which may result in dysfunctional proteins that contribute to disease. This highlights the importance of RNA in the translation of genetic information.
In addition, certain types of non-coding RNAs are significant in the context of genetic disorders. Impairments in these RNAs can lead to conditions like Prader-Willi syndrome or Angelman syndrome, illustrating how alterations in RNA processing can have profound effects.
Efforts in gene therapy focus on correcting these issues by targeting RNA directly. This capability represents a promising avenue for restoring normal function, thereby providing potential cures for previously untreatable disorders.
Viral RNA and Pathogenesis
Viral RNA is central to the life cycle of many pathogens. Viruses, such as influenza and SARS-CoV-2, rely on their RNA to hijack the host cellular machinery, leading to infection and disease. The interaction of viral RNA with host RNA can unravel important insights into viral pathogenesis and immune escape.
Research on RNA-based antiviral strategies has gained momentum. By targeting viral RNA, researchers hope to develop new therapeutic agents. For instance, RNA interference (RNAi) offers a method to silence viral genes, thereby impeding replication.
In summary, RNA's pivotal role in health and disease cannot be overstated. From influencing cancer biology to genetic disorders and viral infections, its implications in contemporary research are vast, paving the way for innovative therapies and health interventions.
"The potential of RNA in understanding and treating diseases is profound. It touches on virtually every aspect of cellular function and regulation."
Technological Advances in RNA Research
Technological advancements in RNA research have transformed the landscape of molecular biology over the past few decades. These innovations provide powerful tools that enhance our understanding of RNA's multifaceted roles in cellular processes. The significance of these technologies cannot be overstated. They allow scientists to explore RNA biology with unprecedented precision and depth. This section highlights key advancements, focusing on their benefits and implications for future research.
Next-Generation Sequencing
Next-Generation Sequencing (NGS) has fundamentally changed how researchers study RNA. This highly efficient technology enables rapid sequencing of RNA molecules, providing an expansive view of the transcriptome. With NGS, researchers can analyze thousands of RNA transcripts simultaneously. This capability has led to significant findings in gene expression patterns, alternative splicing, and RNA stability.
One noteworthy application of NGS is its role in discovering novel RNA species, such as non-coding RNAs. These previously underexplored molecules are now recognized for their crucial roles in regulating gene expression and cellular functions. Moreover, NGS facilitates personalized medicine by allowing the examination of patient-specific RNA profiles. This paves the way for tailored treatments in various diseases.
The rise of NGS has not only increased the scale of RNA studies but also their accuracy. Traditional methods often required extensive time and resources. NGS, on the other hand, drastically reduces the time from sample collection to data analysis. This efficiency accelerates research timelines, allowing for rapid iterations and validations of hypotheses.
CRISPR and RNA Editing
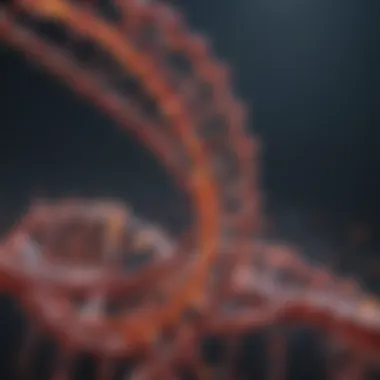
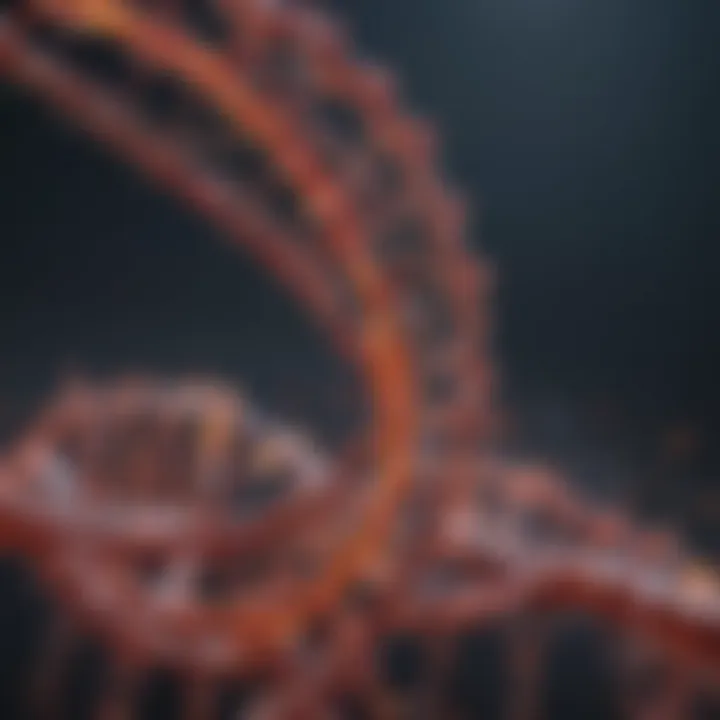
The advent of CRISPR technology has introduced groundbreaking possibilities for RNA editing. Unlike conventional gene editing, which modifies DNA, CRISPR allows for precise alterations of RNA molecules. This capability enables researchers to investigate the functional consequences of specific RNA changes.
Using CRISPR for RNA editing has numerous applications in research and medicine. For instance, it can be employed to correct mutations at the RNA level that lead to diseases. This method offers a unique approach to targeting the RNA transcripts associated with specific pathologies, providing new avenues for therapeutic intervention.
Moreover, RNA editing with CRISPR can enhance synthetic biology applications. Researchers can design RNA sequences to produce desired proteins more efficiently, opening new doors in biotechnology. However, ethical considerations around RNA editing technologies must be taken into account. Controlled usage and thorough understanding of potential off-target effects are essential to ensure safety and efficacy in future applications.
Current Challenges in RNA Research
RNA research has made notable progress in recent years, yet it faces several significant challenges that can hinder advancements. Understanding these challenges is crucial, especially as the potential of RNA in therapeutic treatments and molecular biology becomes more evident. This section will elucidate key issues currently influencing RNA research, including the complexity of riboregulation and various technological limitations that researchers encounter.
Riboregulation Complexity
Riboregulation refers to the regulatory mechanisms governing RNA molecules, particularly their synthesis, stability, and translation. The complexity inherent in riboregulation arises from their multifaceted roles within cells. RNA is no longer seen as just a simple messenger; instead, it is involved in intricate networks that control gene expression and cellular responses.
- Diverse RNA Types: Different RNA types, from mRNA to non-coding RNAs, play distinct roles in regulation. For example, microRNAs can inhibit translation, while long non-coding RNAs might scaffold protein complexes. This diverse functionality complicates the understanding of how these molecules cooperate within the cellular environment.
- Post-Transcriptional Modifications: RNA undergoes numerous modifications after transcription. These might include methylation, pseudouridylation, or editing. Each modification potentially alters the RNA's stability and function, adding layers of complexity. Researchers must untangle how these modifications affect overall gene expression and subsequent phenotypes.
- Environmental Influences: The cellular environment can dramatically influence RNA behavior. Factors such as stress, nutrient availability, and signaling pathways can modify RNA function or stability. Capturing the dynamics of these interactions presents significant experimental challenges.
The intricate complexity of riboregulation not only serves as a scientific curiosity but may also hold keys to understanding diseases. The more nuanced our grasp of riboregulation, the more equipped we are to develop targeted therapeutics.
"Understanding riboregulation is essential for harnessing the full potential of RNA in both research and clinical applications."
Technological Limitations
Despite advancements, technical challenges continue to beset RNA research. The following points summarize prominent limitations:
- Detection Sensitivity: Many RNA types exist in low abundance within cells. Techniques that lack the sensitivity to detect these RNAs face challenges in providing a full landscape of gene expression. Current methods may miss vital signals that contribute to biological phenomena.
- RNA Stability: RNA is inherently unstable, degrading quickly in experimental setups. Maintaining RNA integrity during extraction and analysis requires sophisticated protocols. Unanticipated degradation can lead to misleading conclusions.
- Data Analysis: The amounts of data generated by high-throughput sequencing are immense. Analyzing and interpreting this data demand robust bioinformatics tools. The need for improved algorithms that can handle complexity without oversimplification is apparent.
- Cost and Accessibility: Advanced methodologies often come with high costs and require specialized knowledge. This poses limitations for many institutions, particularly those in resource-limited settings, to participate in cutting-edge research.
In summary, addressing current challenges in RNA research is critical for accelerating our understanding of RNA's biological significance. As we advance, solutions to these hurdles will be necessary for the next frontier in RNA biology.
Future Directions in RNA Biology
RNA biology is at the forefront of contemporary scientific research, presenting an array of opportunities for advancing both basic and applied sciences. Understanding how RNA interacts within cellular mechanisms holds great promise for addressing various health issues and is essential for emerging technologies. This section explores two prominent areas under the umbrella of future directions in RNA research.
Therapeutic Interventions
Therapeutic interventions focusing on RNA are gaining momentum due to the intricate roles that various RNA molecules play in regulating gene expression and cellular processes. One significant application is the development of RNA-based drugs that can specifically target disease-causing genes. For instance, antisense oligonucleotides (ASOs) can be designed to bind to specific mRNA sequences, effectively silencing harmful genes responsible for diseases like Duchenne muscular dystrophy.
- Potential benefits include:
- Precision: Targeting specific RNA sequences allows for a tailored approach in therapy, minimizing off-target effects.
- Versatility: RNA therapies have applications across various diseases, from genetic disorders to cancer.
- Reduced toxicity: Compared to traditional small-molecule drugs, RNA-targeted therapies can often exhibit fewer adverse effects.
Despite these advantages, challenges remain in delivery methods and stability of RNA in biological systems. Developing effective delivery systems that ensure the RNA therapeutics reach the target cells intact is crucial. Moreover, optimizing the design of RNA molecules to enhance their stability is necessary for long-term therapeutic efficacy.
Exploring RNA-Based Vaccines
The emergence of RNA vaccines, particularly highlighted by the success of the Pfizer-BioNTech and Moderna COVID-19 vaccines, marks a transformative shift in vaccine technology. RNA-based vaccines utilize mRNA to instruct cells to produce specific proteins that elicit an immune response. The flexibility and speed of designing these vaccines for new pathogens present a significant advantage over traditional vaccines.
- Key aspects to consider include:
- Rapid Development: RNA vaccines can be designed and produced much quicker than conventional methods, enabling swift responses to emerging infectious diseases.
- Robust Immune Responses: These vaccines have shown the potential to generate strong and lasting immunity against various pathogens.
- Easy Adaptation: Modifying RNA sequences to tackle new variants or strains of a virus can be done without extensive changes to production processes.
"The capability of RNA to serve as both a messenger and therapeutic target opens new avenues for biomedical research, enhancing our understanding of complex biological systems."
In summary, the importance of future directions in RNA biology cannot be overstated. As we look forward, the ongoing investment in this area promises not only to yield novel therapeutics and vaccines but also to deepen our fundamental knowledge of RNAβs multifaceted roles in biology.
Epilogue
In this article, we have explored the wide-ranging implications of RNA biology in contemporary research. RNA is not merely a messenger of genetic information. It is a dynamic molecule central to numerous biological processes. The understanding of RNA has shifted dramatically in recent years. The significance of RNA is underscored by its roles in various diseases and therapeutic applications.
Synthesis of Insights
The analysis has highlighted several key points about RNA's multifaceted roles:
- RNA's involvement in gene regulation and expression serves as a fundamental aspect of cellular function.
- Recent advancements in technology, such as Next-Generation Sequencing, have enabled researchers to uncover the complexity of RNA networks.
- The applications of RNA research in health, particularly concerning therapeutic interventions and vaccines, present promising avenues for future study.
In synthesizing these insights, it becomes clear that RNA's roles extend far beyond those traditionally recognized. Understanding these roles is crucial for future innovations in medical and biotechnological fields. This emphasis on RNA biology can guide the development of new therapies and enhance our overall understanding of biological systems. As research continues to unfold, the implications of RNA biology will likely influence the direction of biomedical research and applications significantly.