Understanding Voltameters in Electrochemical Analysis
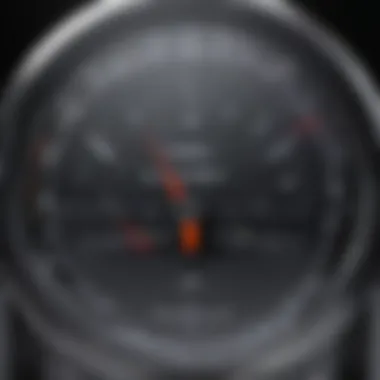
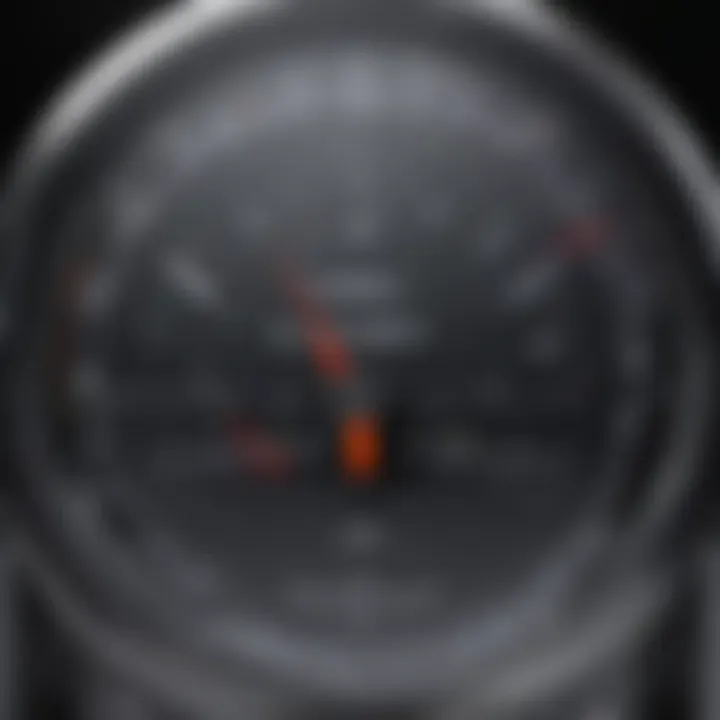
Intro
In the realm of electrochemical analysis, few instruments are as critical as the voltameter. This device is not just a means of measuring the flow of electrical currents, but it embodies the intersection of theoretical principles and practical application. Understanding how voltameters function, as well as the different types available, can greatly enhance the efficiency of experimental setups, leading to more accurate results across various fields, including materials science and bioengineering.
Summary of Objectives
This discussion aims to elucidate the fundamental aspects of voltameters, starting from their basic operational mechanisms to their diverse applications. By jumping into the nitty-gritty of calibration processes and voltametric techniques, we hope to uncover the multilayered operational dynamics of these devices. Ultimately, the goal is to provide a thorough understanding that can serve as a resource for students, researchers, and professionals alike.
Importance of the Research
The significance of this research cannot be understated. As technology advances, the application of voltameters has surged, leading to innovations that further enhance their reliability and scope. For instance, in environmental science, voltameters are essential for detecting pollutants at minute concentrations, vital for assessing the health of ecosystems. Meanwhile, they play a crucial role in electroanalysis within clinical settings, where precision can dictate the success of medical diagnostics. These examples spotlight the profound impact voltameters have across multiple disciplines, underscoring the necessity of mastering their usage and principles.
Understanding Voltameters
In the realm of electrochemical analysis, understanding voltameters is crucial. These instruments play a pivotal role in measuring electrical currents associated with redox reactions. Their ability to deliver precise readings lends itself to various scientific disciplines, which justifies their status as indispensable tools. The fundamental connection between voltage and current charts the course for a wealth of practical applications, from chemical analysis in laboratories to real-time environmental monitoring. This section highlights the key aspects of voltameters, explaining their definitions, functionalities, and historical transformations.
Definition and Functionality
A voltameter can be defined simply as a device that measures the current that flows through an electrolyte solution when a specific voltage is applied. Fundamentally, it operates based on the principles of electrochemistry, where electrical energy prompts chemical reactions. Depending on the voltametric technique used, the device collects data that reveals important characteristics of the electrochemical system under investigation.
The functionality of voltameters is built on their ability to control and measure voltage while simultaneously monitoring the resultant current. They generate voltammograms, which are graphical representations of current against voltage over time. This graphing capability allows researchers to glean insights into reaction mechanisms, diffusion properties, and even the concentration of analytes. Every detail counted on a voltammogram serves as a window into the molecular world, providing depth to our understanding of chemical behaviors.
Key Benefits Include:
- Precision: Voltameters provide high sensitivity and accuracy when measuring low concentrations of substances.
- Versatility: Numerous voltametric techniques cater to different types of chemical analyses, making them applicable across various fields, including environmental science, biochemistry, and industrial applications.
- Real-time Monitoring: Certain voltametric methods allow for continuous analysis, helping in dynamic system exhaustion and faster feedback for experimental adjustments.
To illustrate their importance, think of a detective analyzing a crime scene. Just as every clue unveils another layer of the mystery, every data point from a voltameter brings us closer to understanding the complexities of electrochemical processes.
Historical Development
The journey of voltametry is tied closely to the evolution of electrochemical science. The origins can be traced back to the experiments of Michael Faraday in the early 19th century, where he laid the groundwork for understanding the relationship between electricity and chemical change. His work on electrolysis provided the insights necessary for developing the first rudimentary voltametric measurements.
Fast forward to the late 19th and early 20th centuries, and voltameters underwent significant advancements thanks to innovative minds like Jesse A. Smith and others. They introduced designs that increased measurement precision and expanded operational ranges. Over the years, the scientific community witnessed them morphing from simple apparatuses into sophisticated instruments equipped with automated features and digital interfaces.
The historical development can be divided into notable stages:
- Early Experiments (1800s): Foundation laid by Faradayβs electrolysis theories.
- Mechanization (Late 1800s - Early 1900s): Transition from manual to mechanical systems improving reliability and ease of use.
- Digital Revolution (Late 20th Century): Integration of computer technology enhancing data collection and analysis.
As a product of iterative innovation, voltameters now represent the fusion of historical knowledge and modern technology, making them essential in todayβs scientific investigations.
Types of Voltameters
The section on types of voltameters is teeming with significance as it lays the groundwork for understanding the diverse applications and functionalities offered by these devices. Voltameters can be categorized primarily into three types: static, dynamic, and differential. Each type brings its unique attributes to the table, tailored to tackle specific challenges in electrochemical analysis. Recognizing these distinctions is crucial for selecting the appropriate voltameter for a given research application.
Static Voltameters
Static voltameters are designed to maintain a constant potential while measuring a resultant current. This type plays a foundational role, particularly useful in studying systems where the environment remains stable. Steel yourself to appreciate their straightforward nature; often, they excel in quantifying analytes at rest, delivering precise measurements crucial for foundational research endeavors.
The mechanics behind static voltameters allows them to influence electrochemical processes without additional complications. Here are a few nuanced points about their operation:
- Stability: The constant potential leads to reproducible results, earning them favor in quality control environments.
- Simplicity: These devices often require less complex setups and can be user-friendly, making them suitable for educators and students alike.
- Ideal Use Cases: Static voltameters shine in applications like environmental testing, where sample conditions typically maintain a degree of constancy.
Dynamic Voltameters
When the tide turns and substances undergo change, dynamic voltameters ride the wave. This type is engineered to adapt to varying conditions, adjusting the potential applied to electrodes, allowing real-time monitoring of reaction rates and other critical parameters. The interplay of time and current sets dynamic voltameters apart, facilitating experiments that demand intricacy.
Consider the following aspects:
- Flexibility: They can map out the kinetics of electrochemical reactions, revealing precious details like diffusion coefficients and reaction orders.
- Versatility: Dynamic voltameters are employed across diverse fields, from pharmaceuticals to materials science, accommodating a range of research needs.
- Real-Time Data: Instantaneous measurements mean that researchers can detect and record changes as they occur, fostering a more profound understanding of reaction pathways.
Differential Voltameters
Differential voltameters adopt a more nuanced approach by comparing currents through two different electrochemical cells or conditions at any given potential. This comparative method is crucial for elucidating subtle chemical behaviors that standard designs may overlook. By assessing variables side-by-side, differential voltameters unveil intricacies within voltametric data that enhance analytical depth.
Key highlights include:
- Enhanced Sensitivity: These devices can effectively differentiate small variations in current, adding layers of understanding to chemical analyses.
- Methodological Rigor: By implementing a dual-cell system, researchers can cross-reference results, adding a level of scrutiny that strengthens findings.
- Application Scope: Differential voltameters are particularly valuable in studies involving trace metals or complex enzyme kinetics, where precision is paramount.
Understanding these three types of voltameters equips researchers, educators, and students to select the right tool for their specific needs seamlessly.##
"Getting the right voltameter is like having the right key for a lock; it opens doors to new discoveries."
From dissecting methodologies to contemplating future upgrades, this section sets the stage for comprehending the multilayered world of voltametry. The knowledge gleaned here invariably aids in making informed decisions regarding experimental design and analytical strategies.
Principles of Voltametry
Understanding the principles of voltametry is like having a roadmap through the complex landscape of electrochemical analysis. At its core, voltametry offers a methodology for assessing the characteristics of chemical species in solution, primarily by examining the relationship between current and voltage. This relationship allows scientists to draw inferences about the chemical processes occurring in the solution, providing invaluable insights not just into the concentrations of different species, but also into their reactivity and thermodynamic properties.
Electrochemical Foundations
The electrochemical foundations of voltametry rest upon fundamental principles surrounding oxidation and reduction reactions. Each chemical species can either gain or lose electrons, and the nature of these transitions is captured through electrochemical cells, which convert chemical energy into electrical energy (or vice versa).
Key components of the electrochemical foundation include:
- The Electrolytic Environment: The choice of the solvent and the supporting electrolyte can dramatically influence experimental outcomes. For instance, using a phosphate buffer solution can stabilize pH levels during an analysis, allowing for more reproducible results.
- The Electrode Reaction: The interaction of the solution with the electrodes sets the stage for measuring current responses, leading to detailed electrochemical behaviors that can be analyzed.
Powerful techniques like cyclic voltammetry exploit this foundational theory by sweeping voltage at varying rates and observing the resultant currents. The peak shapes and locations on a voltammogram can tell researchers a lot about the electron transfer dynamics going on under the surface.
"Electrochemistry is where chemistry meets physics - a perfect blend for understanding molecular nuances."
Current-Voltage Relationship
The current-voltage relationship in voltammetry is critical for discernment in analysis. The relationship often manifests as a polarogram or voltammogram, graphical representations that depict how far current changes as voltage is adjusted.
Essential factors influencing this relationship include:
- Scan Rate: A faster scan rate may lead to increased peak currents, offering insights into reaction kinetics. Conversely, slow scan rates can result in shifts of peak potentials, revealing details about equilibrium states.
- Concentration of Analytes: The higher the concentration, the higher the current response, due to more species participating in the electrochemical reaction at any given moment.
- Temperature and Pressure: Variations can affect the kinetics and thermodynamics of the electrochemical reactions, thus influencing the current response as well.
A common formula information is:
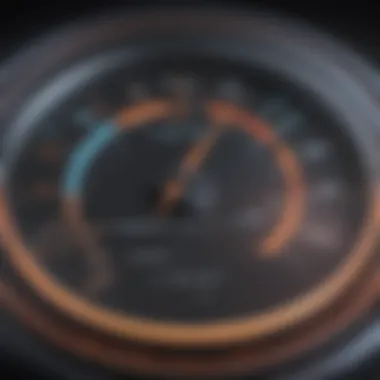
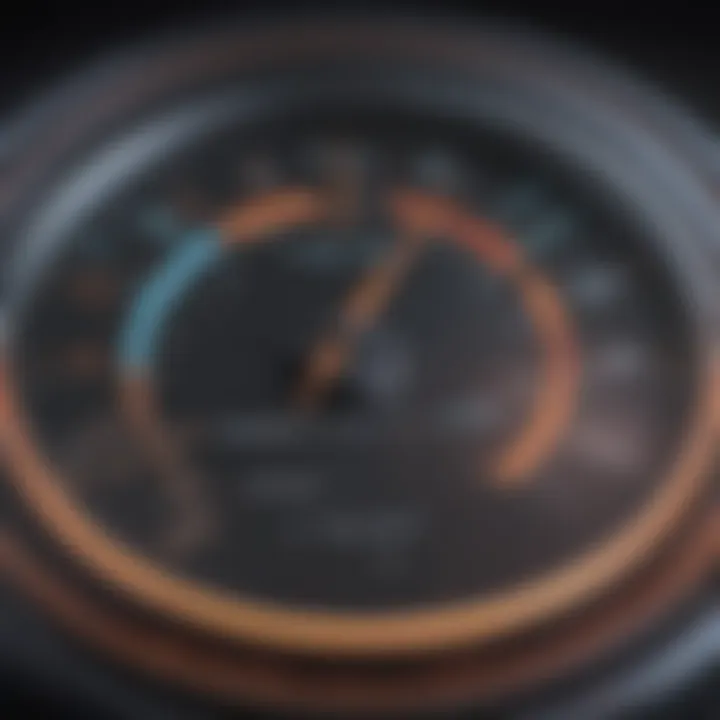
Where:
- I: The current
- Iβ: The limiting current
- Ξ±: The charge transfer coefficient
- n: Number of electrons exchanged
- F: Faraday's constant
- E: The applied potential
- Eβ: The standard potential
- R: Universal gas constant
- T: Absolute temperature
This relationship emphasizes how subtle shifts in voltage can lead to substantial variations in current, exemplifying the sensitivity that voltammetry offers in electrochemical exploration.
In summary, recognizing the principles behind voltametry equips researchers and students alike with essential tools for navigating the intricate details of electrochemical analysis, transforming observations into actionable data.
Equipment and Components
The effective operation of voltameters greatly relies on the equipment and components that form their foundation. Understanding these elements is vital for anyone wishing to delve deeper into electrochemical analysis. Each component plays a unique role, contributing to the voltameter's overall performance and accuracy. This section will explore the main components, namely electrodes, reference electrodes, and power sources, illustrating their significance in the voltametric processes.
Electrodes
Electrodes are the heart of any voltametric setup. They serve as the interface for the electrochemical reactions that occur in the solution. The anode and cathode are two primary types of electrodes utilized in various voltammetric methods. Choosing the right electrode material is crucial for accurate measurements. Common materials include platinum, gold, and glassy carbon, each offering distinct benefits depending on the experimental context.
For instance, platinum electrodes are known for their excellent conductivity and resistance to corrosion, making them suitable for a wide range of chemicals. On the flip side, glassy carbon electrodes are often favored for their low background current and ability to modify surfaces for selective reactions. In short, the material and design of an electrode can significantly influence sensitivity and range of detection in voltametric analysis.
Furthermore, the surface morphology of the electrodes can affect the kinetics of the electrochemical reactions. Roughened surfaces can offer a greater surface area, enhancing the reaction rates and providing better analytical performance.
Reference Electrodes
The stability and accuracy of voltametric measurements hinge on the reference electrodes. These electrodes maintain a constant potential against which other potentials are measured. Common types of reference electrodes include the silver/silver chloride (Ag/AgCl) electrode and the saturated calomel electrode (SCE).
Using a reliable reference electrode is essential for maintaining the integrity of the electrochemical data. The choice of reference electrode not only impacts the reproducibility of results but also influences the compatibility with different solution environments.
Key considerations when choosing a reference electrode include:
- Stability: Should maintain a reliable potential over time.
- Reproducibility: Should provide consistent voltage readings across multiple experiments.
- Compatibility: Must be suitable for the specific electrochemical environment used in the analysis.
By ensuring that the reference electrode meets these considerations, researchers can significantly minimize measurement errors tied to fluctuations in potentials during experiments.
Power Sources
Power sources provide the necessary potential for driving the electrochemical reactions occurring in the voltametric cell. The type of power source can significantly impact the quality of data obtained from experiments. In many instances, high-performance power supplies are utilized to ensure accurate control of potential.
Considerations when selecting power sources include:
- Stability of output: A stable potential output ensures reliable data over time.
- Voltage range: Should cover the range of potentials needed for the experiments.
- Current capacity: Must match the demands of the electrochemical cells.
A constant current source can also be employed, which might be beneficial for some dynamic voltametric techniques. Depending on the experimental design, different configurations may be needed regarding circuit design and output regulation.
Applications in Scientific Research
The role of voltameters in various fields of scientific research cannot be overstated. They serve as critical tools for accessing a multitude of electrochemical processes. From accurate measurements in chemical analysis to monitoring environmental pollutants, voltameters enable scientists to extract valuable data that shapes our understanding of chemical interactions and biological systems. This section delves into several key applications, illustrating how scientists leverage these devices to advance their inquiries and findings.
Chemical Analysis
Voltameters are vital in chemical analysis as they facilitate the detection and quantification of substances in solution. Their operation hinges on measuring the current response of an analyte under a controlled voltage. Researchers often use techniques like cyclic voltammetry, which provides insight into redox properties and reaction kinetics.
- Detection Limits: One of the standout features of voltametric techniques is their ability to achieve low detection limits, making them suitable for trace analysis. This is especially important when working with precious or hazardous materials, where every microgram counts.
- Selectivity: Through modifications in method parameters or electrode materials, scientists can enhance selectivity for specific compounds, minimizing interference from other species present in a sample. This capability is crucial when analyzing complex mixtures, such as in the case of pharmaceutical formulations or biological fluids.
In various sectors such as pharmaceuticals, agronomy, or material science, voltameters have slotted in as preferred choices for obtaining qualitative and quantitative data efficiently and precisely.
Biochemical Applications
In the realm of biochemistry, voltameters are used extensively for detecting biomolecules, studying enzymatic reactions, and monitoring cellular activities. They offer unique advantages due to their sensitivity and ability to operate in dynamic systems.
For example, researchers have successfully employed enzyme-based biosensors using voltametric methods to detect glucose levels in diabetes management. The specificity of these sensors comes from the enzyme's innate capability to bind to its target, yielding an electrical signal proportional to its concentration.
- Real-Time Monitoring: Another compelling aspect of biochemical applications is the potential for real-time monitoring of metabolic processes. This capability aids in understanding physiological changes that occur under stress or disease conditions.
- Point of Care Testing: The portability and user-friendliness of specific voltametric devices have made them invaluable for point-of-care diagnostics, allowing for quick results outside of conventional laboratory settings.
Environmental Monitoring
Voltameters are increasingly employed in environmental monitoring to assess pollution levels and identify contaminants in water and soil systems. The ability to provide rapid, on-site measurements has transformed environmental assessments.
- Heavy Metal Detection: For instance, voltametric techniques are commonly used to monitor heavy metal concentrations in wastewater systems. The impact of heavy metals on human health and ecosystems can be profound, making these measurements crucial.
- Pollutant Profiling: By analyzing the voltage response of various contaminants, researchers can establish comprehensive profiles of pollutants, leading to informed decisions regarding environmental protection measures.
In summary, the applications of voltameters span critical fields of research, offering tools that lead to enhanced analytical capabilities. The interplay between voltametry and scientific inquiry continues to evolve, presenting new opportunities for innovation and discovery.
"Voltameters are not just instruments; they are gateways to understanding the molecular world."
Their expansion into diverse applications illustrates their significance, paving the way for further advancements in research methodology and environmental stewardship.
Preparation of Solutions
In the realm of voltammetry, the preparation of solutions stands as a pivotal element that can dramatically influence experimental outcomes. Itβs not merely a matter of mixing chemicals; it's about crafting the right milieu that can facilitate effective electrochemical reactions. The precision in preparing these solutions directly correlates to the quality of data one can gather, making it all the more critical for researchers and practitioners alike.
Reagent Selection
Choosing the correct reagents is paramount in developing solutions for voltammetric analysis. Every reagent possesses unique properties, and their interactions can yield varying results. For instance, if one were to select potassium chloride for a specific application, its ionic strength can significantly affect conductivity and, subsequently, the voltammetric response. It's wise to consider:
- Purity: High-purity reagents minimize contaminants that could lead to erroneous data.
- Concentration: This should align with the desired detection limits and sensitivity requirements of the voltametry method.
- State: Decide between solid, liquid, or gas phase reagents as each has particular demands for handling and measurement.
Ultimately, the chosen reagents ought to complement the analysis goals while fitting into the broader context of method suitability.
Concentration Calculations
Calculating concentrations accurately is an art form in voltammetric work, fundamentally tied to the reliability of results. Concentration affects the response current in voltammetry, as dictated by the laws of electrochemistry. A clear understanding of molarity, molality, and normality ensures that the solution behaves as expected during experimentation. Researchers often utilize formulas to convert stock solutions into the desired concentrations. For instance:
[ C_1V_1 = C_2V_2 ]
where:
- (C_1) is the concentration of the stock solution,
- (V_1) is the volume of the stock solution,
- (C_2) is the concentration needed, and
- (V_2) is the final volume of the diluted solution.
Accuracy in these calculations cannot be overstated, as even minor miscalculations could lead to significant deviations in analytical results, often skewing data or producing unreliable graphs.
Solution Stability
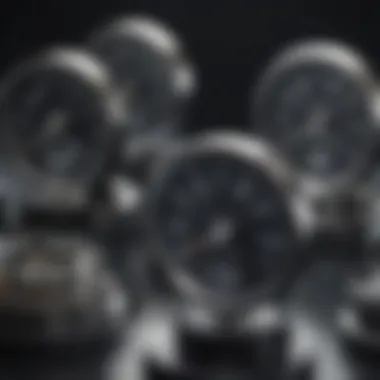
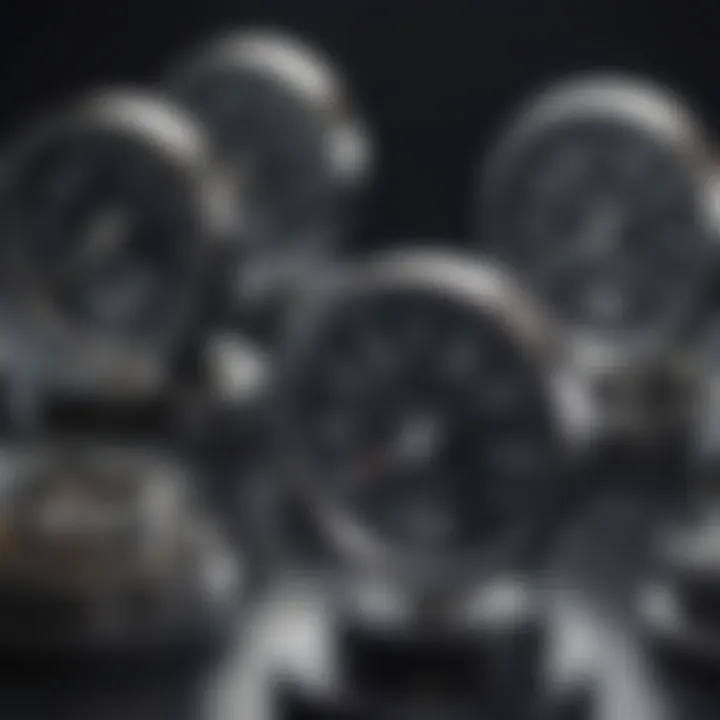
Once prepared, the stability of the solution must be scrutinized. A solution's stability defines how long it can retain its properties without undergoing changes that could impact voltammetric measurements. Factors influencing this stability include temperature, light exposure, and pH levels. For example:
- Precipitation: Some solution components may precipitate if left to sit for too long, altering the concentration and the expected voltammetric reaction.
- Volatility: Certain solvents can evaporate over time, leading to concentration changes; methanol is a classic example where loss through evaporation can become problematic, particularly at elevated temperatures.
- Microbial Growth: Some aqueous solutions can become breeding grounds for bacteria, which not only alters concentration but can also introduce unknown variables into the analysis.
To mitigate these risks, itβs recommended to prepare fresh solutions for critical experiments and store any exceeding volume in a stable, dark environment at controlled temperatures to prolong its usability.
"The preparation of solutions is not simply a step in protocol; itβs the foundation of successful electrochemical analysis. Without careful consideration, even the best-designed experiments can falter at this stage."
Thus, by diligently selecting reagents, accurately calculating concentrations, and ensuring solutions are stable, researchers can significantly enhance the reliability and validity of results in voltammetric studies.
Calibration Techniques
Calibration techniques are a cornerstone in ensuring the reliability and accuracy of voltametric measurements. When dealing with the nuanced interactions in electrochemical analysis, the precision of the data collected can significantly sway the interpretations drawn from experimental results. Not adhering to proper calibration can lead to unreliable readings, which consequently might mislead subsequent research and application efforts. In the realm of voltametry, calibration serves not just as a quality control measure but also as a pathway to harmonize the equipment with the electrochemical principles at play.
Standardization Procedures
Standardization is the process of ensuring that measurement outcomes align with established references. This can be achieved through the use of standard solutions which have known concentrations of the analyte of interest. The fundamental goal here is to produce a comparison point that can validate the voltameter's functionality under specific conditions.
To effectively standardize a voltametric setup, several steps should be adhered to:
- Preparation of Standard Solutions: Standard solutions must be prepared with care, utilizing reagents of high purity and following prescribed techniques to avoid contamination or inaccuracies.
- Electrode Condition Assessment: Before any analysis, electrodes should be prepped and assessed to ensure they are free from residues and responsive to given voltages.
- Stepwise Current Application: Employ a range of current applications to ascertain if the response from the voltameter aligns coherently with expected results. An irregular response may indicate issues with the electrode or the device needed for adjustment.
- Documentation of Results: Meticulously document all readings obtained during the calibration phase. This forms a foundation for any adjustments needed and aids in establishing historical data for future references.
By diligently following standardization procedures, one ensures that the voltametric measurements remain accurate over time, bolstering the reliability of the analytical results derived during experiments.
Error Minimization Strategies
The quest to diminished error in voltametric measurements is ongoing. There are several strategies one can employ to enhance precision and reduce sources of inaccuracies.
- Minimize Environmental Influences: Temperature fluctuations or electromagnetic interference can skew results. Conducting experiments in controlled environments is essential.
- Calibration Frequency: More frequent calibration checks help identify any drifts in performance early on, saving time and resources in the long run.
- Consistent Protocols: Establishing standardized operational protocols for experiments promotes uniformity. This consistency can limit variables that could otherwise influence results.
- Utilization of Quality Reagents: Using high-grade, pure reagents minimizes the chance of impurities affecting the outcome. Inconsistent reagent quality can lead to significant variability.
- Cross-Checking Results: Incorporating a second method of analysis as a benchmark allows for cross-validation of results. This multi-faceted approach strengthens confidence in the data.
Each of these strategies plays a vital role in promoting precision in voltametric analysis. By capitalizing on a mix of proper techniques and thoughtful planning, researchers can enhance the quality of the data gathered, leading to more substantial insights in their work.
"Accurate calibration techniques are the bedrock of trustworthy voltametric analysis, ultimately ensuring that scientific inquiry does not veer off course."
The significance of calibration techniques cannot be overstated in the realm of voltametry; they are essential not only for producing reliable data but also for elevating the overall standard of electrochemical research.
Limitations of Voltameters
Understanding the limitations of voltameters is just as crucial as recognizing their capabilities. By comprehensively grasping these constraints, researchers and practitioners can navigate challenges in electrochemical analyses more effectively. While voltameters are vital tools in electrochemistry, they are not without their drawbacks, which can impact readings and interpretations of data significantly. Below, we delve into two noteworthy limitations: sensitivity issues and interference from external factors.
Sensitivity Issues
Voltameters, particularly those used in analytical chemistry, demand a high degree of sensitivity to detect low concentrations of analytes. However, achieving this level of sensitivity can sometimes be problematic. For example, in measuring trace elements or metabolites, the current responses may be too low to register substantial readings, leading to potential misinterpretations.
The following points highlight some sensitivity concerns:
- Noise Interference: In a laboratory setting, various sources of electronic noise can distort signals. This interference might stem from nearby equipment or even fluctuations in power supply. Hence, what seems like a definitive reading might mask real variations in current.
- Matrix Effects: The presence of complex matrices, like biological samples, can complicate sensitivity assessments. Different components in a solution can absorb signals, contributing to lower sensitivity in the detection process.
- Electrode Surface Condition: The state of the electrode surface plays a significant role in sensitivity. If electrodes are not adequately maintained or coated, they may present suboptimal responses, limiting their ability to detect low-level signals accurately.
Interference from External Factors
The surroundings in which voltametric measurements are carried out can dramatically impact results. External factors such as temperature, pH, and the presence of impuities pose considerable challenges. These elements can not only skew results but also affect reproducibility.
Two major forms of interference present themselves:
- Temperature Variations: Changes in temperature can alter reaction kinetics and solubilities, hence influencing electrochemical processes. For instance, an increase in temperature can enhance reaction rates but may lead to excessive noise, corroding the integrity of data collected.
- Chemical Interference: Competing reactions occurring within the solution can masquerade as the target analyte. For example, if a voltameter is used to analyze a mixture containing various ions, other species may produce currents that overlap with those intended for analysis, leading to confusion in interpretation.
"Being aware of potential interferences allows for better planning and execution of experiments, affording practitioners opportunities to mitigate these issues strategically."
To navigate these limitations, it's recommended to perform preliminary experiments and calibrate carefully. These practices may involve employing advanced calibration techniques, utilizing internal standards, and maintaining alternative testing environments to minimize the impact of such external factors.
Integrating Technology in Voltametry
Integrating technology into voltametry represents a significant leap in how electrochemical analyses are conducted. The modern landscape demands precision, speed, and accuracy, all of which are enhanced through tech advancements. This integration isn't merely about switching from manual to automated systems; it's a holistic change that includes software solutions, automation, and remote monitoring features. Efficiency of the process gets amplified while reducing human error, impacting the overall reliability of experimental results.
Software Innovations
Software plays a pivotal role in the evolution of voltametry. Recent developments have led to programs that not just record data but analyze it in real-time. These innovations serve a multitude of purposes:
- Data Visualization: The ability to see results in dynamic graphs allows researchers to interpret complex data patterns swiftly.
- Integration with Lab Equipment: Modern voltameters can seamlessly communicate with other laboratory apparatus, improving workflow coherence.
- Advanced Algorithms: Todayβs software employs sophisticated algorithms that can predict results based on pre-existing data, assisting in hypothesis formation.
Moreover, tailored software can significantly enhance calibration processes. Researchers can execute more streamlined methods, leading to increased reproducibility across experiments. A researcher can download software updates that reflect the latest industry standards, ensuring compliance and accuracy. This continual evolution means that those relying on voltametry remain at the forefront of technology and methodology.
Automation and Remote Monitoring
Automation in voltametry heralds a new era of efficiency. Automated systems take over repetitive tasks, allowing scientists to focus on analysis and interpretations rather than routine data collection. A typical setup could involve:
- Automated Sample Handling: Reduces the risk of contamination and enhances the accuracy of sample analysis.
- Real-Time Monitoring: Scientists can watch experiments unfold from a distance and make adjustments as necessary without being physically present. This is particularly useful in high-stakes environments where time and accuracy are critical.
βAutomation doesnβt replace the researcher; it enhances their capacity to innovate and discover.β
Remote monitoring is equally crucial, especially in a world where collaborations often span continents. Researchers can access data, adjust parameters, or troubleshoot errors remotely, making oversight easier and leading to faster decision-making processes. This integration not only adds to the efficiency but also empowers teams spread across various locations.
In summary, the integration of technology in voltametry isn't just a convenience; it's a necessity in pushing the boundaries of what we can achieve in electrochemical analysis. By understanding and embracing software innovations and automation, researchers can significantly advance their work and uncover insights that were previously unattainable.
Future Directions in Voltametric Research
The ongoing evolution of voltammetry is vital for its applications across various fields of study. By exploring future directions in this domain, we unlock new methods and refine existing ones, improving upon both versatility and accuracy of voltameters. In an era where precision is gold and time is money, the progression of voltametric research could not be more necessary.
Emerging Techniques
In recent years, the landscape of voltammetry has seen a surge in emerging techniques that promise to revolutionize electrochemical analysis. For instance, the incorporation of nanotechnology into voltammetric methods has led to the development of nanosensors. Such sensors enhance sensitivity, enabling the detection of trace amounts of analytes that traditional methods might overlook.
Given the complexities involved in biochemical detection, these enhanced capabilities are not just beneficialβtheyβre necessary. Automation in data acquisition and processing is another emerging trend. Utilizing machine learning algorithms helps streamline the analysis process, allowing for quicker and more accurate evaluation of electrochemical data. This is a game changer in environments like clinical diagnostics, where decisions often need to be made rapidly.
Moreover, as researchers grapple with more complex samples, techniques like differential pulse voltammetry and square wave voltammetry have garnered attention. These methods deliver finer resolution and can separate overlapping signals, which is particularly useful when analyzing multimodal mixtures.
"Emerging voltammetric techniques are pushing the boundaries of what's possible, opening new doors in research and industrial applications."
Interdisciplinary Approaches
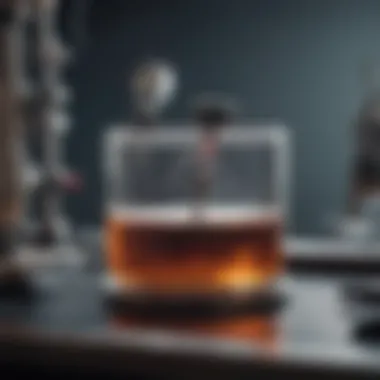
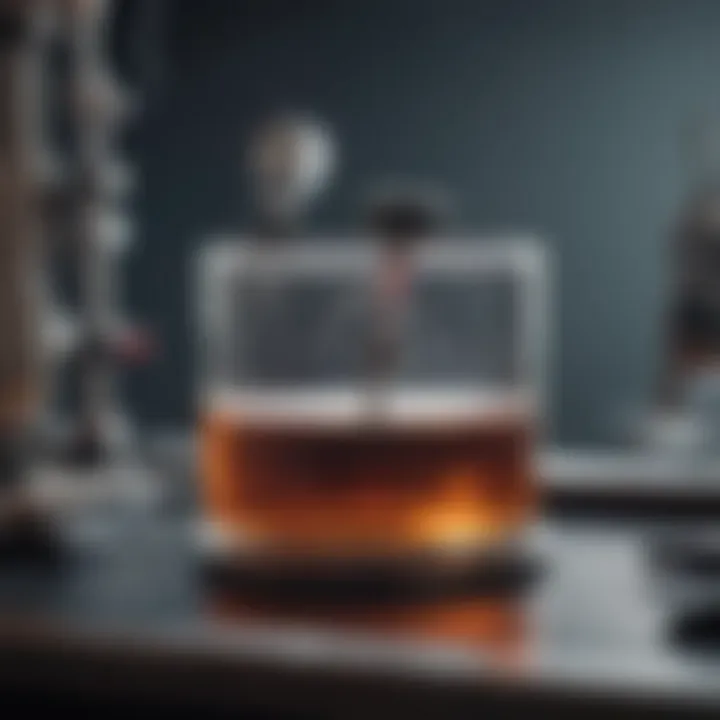
Voltammetry does not exist in a vacuum. By taking an interdisciplinary approach, researchers can tap into various fields such as material science, biology, and environmental science. Collaborations across these disciplines deepen our understanding of voltammetric methods and unveil novel applications.
For instance, the study of electroactive materials, a significant branch of material science, feeds into the development of better electrode designs. These materials can lead to improved performance characteristics in voltammetric analysis, thus enhancing reliability in measuring physiological parameters in biomedical research.
Similarly, in environmental sciences, combining voltammetry with geospatial technologies allows for high-resolution mapping of contaminants, turning a mere measurement tool into a powerful diagnostic instrument. This synergy between disciplines not only amplifies the effectiveness of voltammetry but also cultivates innovation.
By fostering interdisciplinary dialogues, we can unearth new metrics for assessing environmental impacts, enrich biochemical analysis, and explore uncharted territory in both domestic and commercial sectors.
Case Studies of Voltametric Applications
The exploration of voltametric applications offers an insightful glimpse into how these powerful instruments are leveraged across diverse fields. By examining specific case studies, we not only solidify our understanding of voltameters but also illustrate their practical impacts on industry and research. These case studies highlight the versatility and utility of voltametric techniques, making clear why they are indispensable tools in both the laboratory and field settings.
Industrial Applications
In the industrial realm, voltameters play a crucial role in monitoring and controlling processes. One pivotal example can be found in the metallurgical sector, where gold plating processes employ voltametry to ensure consistent thickness and quality of the gold layer.
When companies engage in electroplating, it is vital to measure the electrical current to determine the deposition rate of the metal. By implementing a static voltameter, operators can track this current in real-time, allowing for immediate adjustments to maintain the desired outcome. This not only enhances product quality but also reduces waste, an important consideration in todayβs environmentally conscious market.
Another notable case involves battery manufacturing. Utilizing differential voltametry, manufacturers can assess the performance characteristics of new battery chemistries during the development stage. This involves examining the current responses of materials in various potential windows. Such assessments enable engineers to optimize battery designs to maximize efficiency and longevity, addressing urgent demands in consumer electronics and electric vehicle production.
Key Benefits of Voltametric Applications in Industry:
- Enhanced quality control of products.
- Real-time monitoring of critical process variables.
- Improvement in resource efficiency, reducing waste and cost.
Case Study in Environmental Studies
In the environmental sciences, voltametric methods unravel the complexities of chemical interactions in ecosystems. A poignant example involves the detection of heavy metals in water, a significant concern for public health and safety. Researchers implemented static voltameters to quantify the presence of lead, mercury, and cadmium in river samples.
Through these studies, it was discovered that certain tributaries exhibited alarmingly high concentrations of heavy metals due to nearby industrial runoff. The voltametric analysis not only confirmed the pollution levels but also provided vital information for formulating strategies to remediate contaminated waters. This type of research empowers policymakers to impose stringent regulations on industries, fostering a cleaner environment.
Furthermore, voltametric methods have proven valuable in studying redox reactions in soil samples. These reactions dictate nutrient availability and microbial activity in ecosystems. By employing dynamic voltametry in laboratory conditions, scientists can simulate natural processes and assess how contaminants alter soil chemistry. Such insights are fundamental for developing sustainable agriculture practices.
"The application of voltametry in environmental research aids not just in detection, but also drives policy change and restoration strategies."
Important Considerations in Environmental Studies:
- Identification and quantification of pollutants in natural waters and soils.
- Understanding the dynamics of ecosystem health through redox potential studies.
- Informing effective remediation and conservation efforts.
Educational Implications of Voltametry
The educational implications of voltametry extend far beyond merely teaching students about a set of tools and techniques. Understanding voltametry is crucial for fostering a deeper appreciation of electrochemical analysis and its significance in both research and practical applications. As the field of electrochemical science evolves, it becomes paramount that educational institutions adapt their curricula to incorporate contemporary methodologies, ensuring that students are well-prepared for the challenges they may encounter in their professional lives.
The teaching of voltametry not only imparts essential technical knowledge but also enhances critical thinking and analytical skills. Students learn to interpret data, troubleshoot equipment, and critically evaluate experimental setups. Moreover, this field encourages interdisciplinary collaboration, as concepts of chemistry, physics, and engineering merge, enriching the learning experience.
Teaching Techniques
Effective teaching techniques for voltametry must cater to diverse learning styles and backgrounds. Here are some approaches educators can utilize:
- Hands-On Learning: Practical experiences, such as laboratory sessions where students can operate voltametric devices, help solidify theory through application. Using actual data collections from experiments encourages engagement.
- Problem-Based Learning (PBL): Structuring lessons around real-world problems can enhance motivation. Students could analyze case studies where voltammetry plays a crucial role, such as detecting pollutants in environmental studies.
- Interactive Simulations: With advancements in technology, educators can employ software simulations to allow students to visualize the principles of voltammetry without the constraints of physical materials and equipment. This can be particularly beneficial when resources are limited.
- Collaborative Projects: Group work can foster peer learning. Assigning projects where students design their experiments can encourage critical thinking and communication skills.
Implementing these techniques can instill a sense of curiosity about voltametry, prompting deeper exploration into the subject matter.
Curricular Integration
For voltametry to attain a prominent place within the educational landscape, its integration into existing science curricula is vital. Here are some factors to consider:
- Interdisciplinary Approaches: Integrating voltametric principles with courses in chemistry, biology, and environmental science can offer students a well-rounded perspective. Understanding that voltammetry intersects various fields enhances appreciation for its versatility and relevance.
- Incorporating Current Events: Lessons on voltametry can be enriched by relating real-time developments in science and technology. Highlighting how voltameters are used for drug monitoring or in renewable energy research can make learning more applicable and engaging.
- Guest Lectures and Workshops: Inviting professionals from the field to share their experiences and insights can bridge the gap between theoretical knowledge and practical application.
- Assessment Strategies: Evaluating student understanding through varied assessment methods, including multiple-choice, practical exams, and reflective essays, can provide a comprehensive gauge of their grasp on the subject.
Incorporating voltammetry into educational paradigms can ensure that the next generation of scientists is well-versed in this essential technology, ultimately advancing the field of electrochemical analysis.
Ethical Considerations in Research
In the realm of electrochemical analysis, particularly when dealing with voltameters, ethical considerations cannot be sidelined. As researchers strive to unravel the mysteries of electrochemical reactions and apply voltametric techniques, they must navigate a landscape where integrity, transparency, and accountability are paramount. This section delves into the ethical implications of research in this field, with a focus on data integrity and the responsible use of voltametric data.
Data Integrity
The foundation of any scientific inquiry lies in the reliability and validity of the data collected. Data integrity refers not only to the accuracy of measurements obtained from voltameters but also to the precision with which those measurements are reported. When researchers manipulate or misrepresent data, they not only compromise their own credibility but also potentially mislead peers and the wider scientific community, leading to bizarre conclusions or misguided further research.
To ensure data integrity, researchers must follow rigorous protocols in both experimental design and analysis. Here are several key aspects that support data integrity in voltametric studies:
- Standard Operating Procedures (SOPs): Implementing SOPs ensures consistency and reliability in the methods used.
- Calibration Standards: Regular calibration of voltameters against known standards helps in achieving accurate measurements.
- Transparent Reporting: Clearly documenting methods, results, and any anomalies promotes transparency and allows for reproducibility.
"Research is a journey where every piece of data can lead to new pathways; maintaining integrity is crucial for navigating that journey."
Responsible Use of Voltametric Data
Having obtained reliable data is one thing; using it responsibly is entirely another. The power of voltametric data can lead to significant breakthroughs in fields such as chemical, environmental, or biological research. However, misuse or misinterpretation of this data poses ethical dilemmas that researchers must confront. Responsible use involves not only accurate reporting but also consideration of how the findings may impact society.
Some considerations for the responsible use of voltametric data include:
- Public Communication: Findings must be communicated honestly, avoiding hyperbole that could exaggerate the significance of results.
- Collaboration and Credit: Proper attribution to collaborators and peers encourages a respectful research environment and promotes trust.
- Usage in Technology Development: Ensuring that voltametric data is used ethically in technological advancements means considering potential environmental impacts and societal implications.
Practicing ethical standards in research fosters a culture of respect and integrity. Researchers contribute to a more accurate understanding of electrochemical processes, ensuring that their findings benefit the scientific community and society at large. Ultimately, adhering to these ethical principles not only strengthens the credibility of individual studies but also enhances the overall integrity of scientific research.
Closure
In summary, the conclusion of this article encapsulates the vital role voltameters play in electrochemical analysis. They are not merely devices; they represent a confluence of theory and practice in the scientific realm. By effectively measuring electrical currents during electrochemical reactions, voltameters facilitate deeper understanding across various disciplines.
The invaluable insights provided by voltameters lead to advancements in chemical analysis, biochemical applications, and environmental monitoring. They allow researchers to derive critical data that can affect both theoretical knowledge and practical applications alike. As we examined in the article, precision in measuring small currents becomes fundamental when interpreting complex electrochemical phenomena.
Also, itβs clear that understanding the calibration techniques discussed earlier is paramount. Calibration is the bedrock that ensures measured values reflect reality, thus, fostering data integrity. These intricate procedures safeguard the reliability of findings in scientific research, reinforcing the credibility of the resulting analyses.
Summary of Findings
Through a meticulous exploration of voltameters, several key points arise:
- Types of Voltameters: Understanding static, dynamic, and differential voltameters reveals the various operational methodologies suited to different applications.
- Principles of Voltametry: The principles underpinning these devices highlight the relationship between current and voltage, illuminating the electrochemical processes.
- Calibration and Preparation: The detailed focus on calibration techniques and solution preparation ensures researchers can attain accurate and reliable outcomes, a necessity in scientific investigations.
- Future Directions: Innovations and interdisciplinary approaches signal a promising future for voltametric research, pointing towards enhanced functionalities and wider applications.
In weaving these findings together, it becomes evident that voltameters are foundational tools that cannot be overlooked. They are at the forefront of electrochemical studies, providing critical insights across scientific communities.
Final Thoughts
As we conclude, itβs essential to reflect on the broader implications of voltametry in electrochemical analysis. These devices are not confined to just laboratories; their applications stretch into environmental studies, industrial processes, and health sciences. The ongoing research and technological advancements suggest that voltametric analysis will remain relevant, if not increasingly vital, in the face of complex scientific inquiries.
Further, ethical considerations must be highlighted as researchers leverage voltmetric data for decision-making and policy development. Ensuring data transparency and responsible usage cannot be taken lightly. As the scientific community continues to push the boundaries of what voltameters can achieve, one must remain vigilant about maintaining high standards in research integrity and application.
Ultimately, this exploration reinforces the understanding that voltameters, while often underappreciated, are pivotal in electrochemical analysis. They marry the principles of chemistry with practical inquiry, acting as a bridge between concepts and real-world applications.